
국내외 스모그 챔버 연구동향
Abstract
The smog chamber is a research facility that simulates atmospheric phenomena such as secondary pollution of ozone and aerosols under different atmospheric conditions. It enables the investigation of photochemical reaction processes, yields, and products, as well as physicochemical properties of secondary aerosol. Since the beginning of smog chamber research under the 1950s, experiments mainly on ozone and particle formation have been conducted, depending on the chamber specification and research trends. Smog chamber research in Korea began in the early 2000s and has continued since 2010 due to growing interest in particulate matter pollution. This study covers the domestic and global history of smog chamber research, as well as significant recent research advancements, which provides recommendations for future studies in smog chamber research.
Keywords:
Photochemical reaction mechanism, Photooxidation product, Ozone, Secondary organic aerosol, Precursors, Aerosol yield1. 서 론
대기 중 초미세먼지는 현대사회의 가장 중요한 환경문제 중 하나이다. World Health Organization (WHO, 세계보건기구)에 의해 개발된 Global Burden of Disease (GBD, 세계 질병 부담) 분석에 의하면 2019년 현재 초미세먼지 (PM2.5)에 의해 연간 4백만 명의 사람이 사망하였으며 (Fuller et al., 2022), 국내에서도 1990년부터 2013년까지 연평균 1만 7,000여 명이 PM2.5의 장기노출로 인하여 조기사망한 것으로 추정된다 (Kim et al., 2018). Organisation for Economic Co-operation and Development (OECD, 경제협력개발기구)에 따르면 particulate matter (PM, 입자상 물질)에 의한 조기사망률은 2050년까지 2010년 기준 두 배 이상 증가할 것으로 예측되었다 (OECD, 2012). 1952년 발생한 런던 스모그와 1943년부터 1950년대 발생한 LA 스모그, 급격한 경제성장으로 2010년대 정점을 기록한 베이징 및 중국 대도시들의 초미세먼지 오염은 nitrogen oxides (NOx, 질소산화물), sulfur oxides (SOx, 황산화물), volatile organic compounds (VOCs, 휘발성유기화합물) 등 대기 중 기체상 화학물질의 광화학반응으로부터 발생하는 초미세먼지에 대한 생성 원인과 반응기작, 영향에 대한 과학적 연구를 촉발시켰다 (Zhang et al., 2015). 국내에서도 2010년대 중반 중국으로부터의 장거리이동과 기상조건의 영향으로 초미세먼지 농도가 다소 증가하는 경향을 보였으며 (Kim et al., 2017; Ahmed et al., 2015), 미세먼지에 대한 대중의 위험인식이 증가하여 (Kim et al., 2020) 초미세먼지에 대한 과학적 연구의 필요성이 제기되었다.
이러한 과학적 대기현상 규명 연구에 사용되는 스모그 챔버는 주로 VOCs의 secondary organic aerosols (SOAs, 이차 유기 에어로졸) (Zhang et al., 2015) 및 오존 생성 수율 (yield)과 기여도를 규명하고, 광화학반응 기작 및 이를 통해 생성된 에어로졸의 물리화학적 특성을 규명하는 연구에 활용되어 왔다. 초기 챔버 연구는 주로 광화학반응에 의한 미세먼지의 생성 기작과 yield를 측정하는 것을 목표로 하였고, 현재의 챔버 연구주제는 대기화학 반응 기작뿐 아니라, 유해성 변화, 구름응결핵 영향 등 다양한 분야를 망라하고 있다 (Chu et al., 2022; Lim et al., 2016). 스모그 챔버는 가스상 전구물질과 seed 입자를 주입하는 주입부, 반응이 이루어지는 반응백 (reaction bag), 광조건을 모사하는 광원, 생성물질을 측정하는 측정/분석부, 생성된 가스와 입자가 배출되는 배출구로 구성된다 (Song et al., 2021; Lim et al., 2016; Bae et al., 2003). 그림 1은 KIST의 소형 및 중형 스모그 챔버의 개략도이다.
대기화학반응 모사실험을 위한 반응물은 seed 입자, VOCs, NOx, 산화제의 순서로 주입된다. Seed 입자와 VOCs를 따로 주입하지 않고 엔진배기가스나 연소가스를 직접 주입하기도 한다. Seed 입자는 미세먼지의 주성분인 (NH4)2SO4이 주로 사용되며 (Tajuelo et al., 2019; Lambe et al., 2015), 모사 조건에 따라서 연소 생성물인 검댕입자 (soot)를 사용하거나 (Kuang and Shang, 2020; Kamm et al., 1999), 대기 중 무기입자를 모사하기 위해 사막먼지 (Kanji et al., 2019; Park et al., 2017)나 MnSO4, ZnSO4, FeSO4와 같은 전이금속-황산염 (Chu et al., 2014, 2013), 해염 에어로졸을 모사하기 위해 NaCl을 seed 입자로 사용하였다 (Sem et al., 2022; Beardsley et al., 2013).
VOCs는 인간활동에 의해 배출되는 anthropogenic VOCs (AVOCs, 인간활동 기원 VOCs)와 자연 중 생물에서 배출되는 biogenic VOCs (BVOCs, 생물활동 기원 VOCs)로 나눌 수 있으며, 대기 중 농도가 높고 배출량이 많은 물질이 주 연구대상이 되어 왔다. AVOCs는 대기 중 농도의 40~50%를 차지하는 alkane 및 alkyl류, 나머지 40%를 차지하는 방향족 화합물질에 대한 연구가 진행되었다 (Srivastava et al., 2022). BVOCs 중에서는 배출량이 가장 많은 isoprene와 α-pinene, β-pinene, limonene을 포함한 monoterpenes류 (C10H16), β-caryophyllene과 같은 sesquiterpene (C15H25), 해양생물에서 기원하는 dimethylsulfide (DMS)가 전구물질로 활용되었다 (Xavier et al., 2019). 다양한 전구물질들의 혼합물을 이용하기도 하였는데, 예를 들어, 자연적으로 방출되거나 인위적으로 배출되는 혼합가스를 직접 스모그 챔버에 주입하기도 하였다.
스모그 챔버에서 광화학반응 시 변화하는 화학물질의 농도를 그림 2에 나타내었다. 일반적으로 VOCs와 NOx를 주입한 후 자외선을 조사하여 광화학반응을 개시한다. 이때 주간 대기화학반응에서 가장 중요한 역할을 하는 OH 라디칼이 생성된다.
NOx를 주입하지 않거나, VOCs/NOx 비가 낮아서 광화학반응성이 약한 경우 추가적인 산화제를 투입하여 산화반응을 촉진한다. 산화제로는 H2O2 (Lugo et al., 2022; Ng et al., 2007), HONO (Li et al., 2022; Han and Jang, 2020; Ng et al., 2007), HCHO (Zhang et al., 2018; Chen et al., 2015; Samy and Zielinska, 2010)가 사용된다. OH 라디칼 생성을 위해 methyl nitrite (CH3ONO) (Sato et al., 2019; Hao et al., 2007), isopropyl nitrite (C3H7NO2) (Lamkaddam et al., 2017; Stroud et al., 2004) 등도 사용되었다. CH3ONO와 C3H7NO2로부터 OH 라디칼이 생성되는 반응은 다음과 같다 (Raff and Finlayson-Pitts, 2010; Becker and Wirtz, 1989).
(1) |
(2) |
(3) |
이와 더불어 주요 라디칼인 O3은 직접 주입되기도 하고, Cl 라디칼은 Cl2로부터 생성된다 (Farrugia et al., 2015; Huang et al., 2012).
각국의 연구기관은 스모그 챔버의 특성과 모사하고자 하는 환경에 따라 다양한 대기물리화학 반응과 그 영향을 관찰하는 연구를 수행해왔다. 국내에서도 Korea-United States Air Quality (KORUS-AQ, 한미 대기질 공동조사), 초미세먼지사업단의 융합연구와 맞물려, 한국을 비롯한 동아시아 대기의 물리화학 반응 특징과 초미세먼지 생성 특성을 규명하고자 하는 연구를 수행해왔다. 본 리뷰논문에서는 국내외의 주요 챔버현황과 최근 연구되고 있는 주요 연구주제에 따른 연구동향을 소개하며, 앞으로의 연구전망을 제시하고자 한다.
2. 국내외 스모그 챔버 연구동향
2. 1 국외 스모그 챔버 연구동향
스모그 챔버는 1950년대부터 개발되어 사용되기 시작하였으며, 이는 1940년대에 NOx와 VOCs 간의 광화학반응이 일으킨 LA 스모그의 대기화학적 반응 기작을 정밀하게 규명하기 위한 목적이었다. 이후 미국, 유럽, 중국, 일본 및 캐나다와 호주 등에서 스모그 챔버가 구축되어 현재까지 600여 편 이상의 논문과 보고서가 출판되어 왔다. 스모그 챔버의 크기에 관한 합의된 정의는 없으나, 편의상 부피가 1 m3 이하인 것은 소형 반응기, 1~10 m3 범위인 것은 소형 스모그 챔버, 10~50 m3 범위인 것은 중형 스모그 챔버, 50 m3 이상인 것은 대형 스모그 챔버로 구분하였다. 특히 최근에는 Oxidation Flow Reactor (OFR, 산화 흐름 반응기) (Kang et al., 2007)가 활용되어 소형의 장비로 장소에 구애받지 않는 가속화된 초미세먼지 생성반응 분석이 가능하다. 각국에 구축된 스모그 챔버의 사양과 과거 동향은 여러 스모그 챔버 리뷰논문에 자세히 요약되어 있다 (Chu et al., 2022; Hidy, 2019; Lim et al., 2016). 본 논문에서는 주요 국가 챔버의 역사를 간단히 소개하고, 최근 연구동향을 중심으로 제시하였다.
미국은 가장 빠른 시기인 1950년대에 이미 스모 그 챔버의 개념을 제시하고 최초의 챔버실험을 수행하였다 (Midwest Research Institute) (Morriss et al., 1957). 1960~1970년대 초기의 RASTEC (Rose and Brandt, 1960), International Business Machines Corporation (IBM) (Steading, 1965), Stanford Research Institute (Doyle, 1970), Battelle Memorial Institute의 Columbus Laboratories (Wilson Jr et al., 1971), FMC corporation 챔버에서는 SOx, NOx, 라디칼의 영향과 실시간 측정법 개발, 광원과 혼합, 수분의 역할 등 기초적인 실험을 수행하였다. 이후 대학과 공공기관을 중심으로 챔버를 구축하여 현재까지도 활발한 스모그 챔버 연구를 수행하고 있다.
UNC Chamber
The University of North Carolina at Chapel Hill (UNC, 노스캐롤라이나 대학교)은 1975년 312 m3 (2×150 m3) 크기의 FEP 테플론 필름으로 이루어진 세계 최초의 옥외 챔버를 시작으로, 이후 여러 차례 다양한 크기의 옥외 챔버를 구축하였다. 이 챔버는 1970년 초 반부터 실험을 시작하여 최근까지도 다양한 연구를 수행하였으며, 특히 챔버 연구의 경향을 단편적으로 보여주는 챔버이다. 초기 연구는 NOx, VOCs의 광화학과정과 광원, 습도의 영향 등 기초 연구가 주를 이루었으며 (Kamens et al., 1981; Jeffries et al., 1976a, 1976b, 1975), 1980~90년대에는 주로 배출가스와 polycyclic aromatic hydrocarbons (다환방향족탄화수소, PAHs) (Kamens et al., 1986), dioxin (Lutes et al., 1992), siloxane (Chandramouli and Kamens, 2001; Latimer et al., 1998)과 같이 인간활동에 의해 발생되는 유해 물질에 산화실험과 기체-입자상 분배, 대기 중 안정성, 독성영향실험이 수행되었다. 2000년대 이후 SOAs 연구가 본격화되면서 β-caryopyllen (Jaoui et al., 2003), α-cedrene (Jaoui et al., 2004), α-pinene (Lee et al., 2004), α-limonene (Leungsakul et al., 2005), isoprene (Chandramouli and Kamens, 2001) 등 다양한 terpene류와 도시대기 중 단일 및 혼합 VOCs (Ebersviller et al., 2012; Kamens et al., 2011; Zhou et al., 2011)의 광화학반응과 생성물의 환경거동 및 생물학적 영향을 연구하였다.
2015년 이후 SOAs의 Oxidation potential (OP)과 독성에 대한 연구 (Hazari et al., 2018; Kramer et al., 2016)와 epoxyldiol-derived sulfated/nonsulfated oligomer 등 ozonolysis 생성물의 정밀 정성분석과 대기화학적 역할에 대한 연구 (Armstrong et al., 2022; Riva et al., 2016)가 수행되어 왔다.
UCR Chamber
University of California, Riverside (UCR, 캘리포니아 대학교 리버사이드)에는 U.S. Environmental Protection Agency (US-EPA, 미국 환경청)의 지원으로 OTC (Outdoor Teflon Chamber), ITC (Indoor Teflon Chamber), ETC (Ernie’s Teflon Chamber) 등 다양한 크기의 실내외 챔버가 구축되어 1970년대 초반부터 도시대기의 광화학반응에 대한 지속적인 연구를 수행하였다. 2005년에는 90 m3 크기의 듀얼 실내 챔버인 EPA-UCR 챔버가 구축되었으며, 실제 대기환경 모사에 적합한 저농도 NOx 실험에 활용되고 있다 (Carter et al., 2005). UCR 챔버에서는 주로 VOCs의 광화학 반응기작과 반응속도 규명을 비롯하여 반응 메커니즘을 규명하고, 이를 대기화학 모델링에 활용할 수 있는 데이터베이스를 구축하였다 (Carter et al., 2012; Dodge, 2000; Carter and Lurmann, 1991; Pitts Jr, 1977; Pitts Jr et al., 1977).
최근에도 camphene (Li et al., 2022), furan (Jiang et al., 2020)과 같은 화학물질의 광화학반응 메커니즘을 규명하여 모니터링-모델링 데이터 간의 비교연구를 수행하였으며, 실제 도시 대기환경에서 발생하는 SOAs 생성 영향인자 연구 (Zhao et al., 2019), AVOCs와 생활화학물질 및 디젤 증기로부터의 SOAs/오존 생성 반응연구 (Li et al., 2018; Li and Cocker, 2018)를 수행하였다.
Caltech Chamber
California Institute of Technology (Caltech, 캘리포니아공과대학교)에는 1985년 65 m3의 옥외 챔버가 구축되어 실험을 수행해왔으며 (Leone et al., 1985), 2001년 28 m3 크기의 FEP 테플론 듀얼 실내 챔버를 구축하였다 (Cocker et al., 2001). 다양한 종류의 monoaromatics (Ng et al., 2007), terpenes (Lee et al., 2006a, 2006b), 12C-alkane (Yee et al., 2013), cycloalkenes (Keywood et al., 2004), α-pinene (Eddingsaas et al., 2012a, 2012b)의 SOA yield를 보고하였으며, 해양에서 생물기원 Iodine으로부터 형성되는 해양 에어로졸 생성 기작 (O’Dowd et al., 2002)을 연구하기도 하였다. CIMS (Chemical ionization mass spectrometry)를 활용하여 isoprene으로부터 Organic nitrate와 epoxide가 생성됨을 관찰하여 보고하였으며 (Paulot et al., 2009a, 2009b), HR-AMS (High-Resolution Aerosol Mass Spectrometer)를 이용한 에어로졸의 화학조성 연구를 도입하기도 하였다 (Chhabra et al., 2010). 특히 이 챔버에서는 wall-loss 현상 (Loza et al., 2010)과 wall deposition (Zhang et al., 2015), vapor-wall transport (Huang et al., 2018), 입자의 전하가 끼치는 영향 (Charan et al., 2018) 등 챔버 실험의 원리 등을 정밀하게 규명함으로써, 챔버 실험과 실제 대기환경 간의 간극을 줄이는 데 필요한 지식을 제공하였다.
UF-APHOR
2011년에 구축된 플로리다 대학교의 University of Florida-The Atmospheric PHotochemical Outdoor Reactor (UF-APHOR)는 2×52 m3의 FEP 테플론 필름으로 이루어진 옥외 챔버로 매우 독특한 주제들을 다루고 있다. 예를 들어, sea salt aerosol (Sem et al., 2022; Beardsley et al., 2013)과 mineral dust (Yu and Jang, 2018; Park et al., 2017; Park and Jang, 2016)를 seed 입자로 사용하여 heterogenous 반응을 연구하였고, 녹조와 적조에서 배출되는 microcystin (Jang et al., 2020)과 Brevetoxin (Sem et al., 2022)의 대기 중 안정성과 유해성 변화에 관한 연구를 수행하였다. 플로리다주는 북아프리카 사하라 사막에서 비산한 사막 먼지가 대서양을 건너 직접적으로 도달하는 지역이며, 해양에서 유입된 해염 에어로졸을 통해 적조 독소가 대기 중에서 검출된 바 있다. 2016년과 2018년에는 심각한 녹조가 발생하기도 하였다. 이 챔버의 연구 결과는 국내의 황사, 녹조현상에 의한 조류독소의 대기화학 반응을 보여준다는 점에서 특기할 만하다.
유럽은 1980년대 초 Wuppertal 대학에서 최초의 챔버 실험을 수행하였으나 규모는 소형반응기에 그쳤다 (Barnes et al., 1983, 1982). 1990년대 중반 본격적으로 독일과 스페인, 영국, 스위스, 프랑스 등 각국에 각기 다른 유형의 중대형 스모그 챔버를 구축하였으며, EUROCHAMP 2020 (https://www.eurochamp.org)라는 협력네트워크를 구성하여 운영하고 있다. 각 챔버의 특성에 따라 다양한 연구를 수행하며, 모사 챔버와 분석기기를 교정하는 검교정 챔버를 운영하고 있다.
EUPHORE
EUPHORE (European PHOtoREactor)는 1998년 스페인 발렌시아에 구축된, 170 m3 크기의 PEF 테플론 재질의 유럽 최초의 대형 옥외 챔버이다 (Klotz et al., 1998). 이 챔버에서는 구축 초기부터 monoterpenes (Hallquist et al., 1999), alkenes (Siese et al., 2001), allyl acetate (Picquet-Varrault et al., 2002)의 SOAs 생성 메커니즘 연구 (Bloss et al., 2005a, 2005b)를 수행해왔다. EUPHORE에서는 LIF (laser induced fluorescence)를 이용한 OH 라디칼 측정법을 검증하고 (Bloss et al., 2004), 챔버 벽면에서 생성된 HONO의 OH 라디칼 생성속도를 규명하여 (Zádor et al., 2006), 이를 바탕으로 다양한 물질의 photolysis 실험을 수행하였다. 2010년대 이후 ethalfluralin herbicide (Muñoz et al., 2014b), chlopyrifos (Muñoz et al., 2014a), lindane (Vera et al., 2015), organothiophosphate insecticidide (Muñoz et al., 2018), thiocabamate herbicideds (Borrás et al., 2017) 등 다양한 살생물제의 OH 라디칼 반응 연구를 통해 규제정책에 활용할 수 있는 환경 중 잔류성과 메커니즘에 관해 연구하였다.
AIDAc
독일 Karlsruher Institut für Technologie (KIT, 카를스루에 공과대학교)에 설치된 AIDAc (Aerosol Interactions and Dynamics in the Atmosphere, classical version)는 용적 84 m3의 알루미늄 합금 (AlMg3)의 수직 원통형 대형 실내 챔버로, 온도는 -90°C부터 +60°C, 압력은 0.01 hPa부터 1,000 hPa까지 조절할 수 있는 챔버이다 (Bunz et al., 1996). 저온/저압 조건까지 모사가 가능하여 대류권의 대기화학반응뿐 아니라 상층 대류권 및 성층권에서의 구름 생성을 모사할 수 있기 때문에 구름 챔버로 불리기도 한다. 각기 다른 파장의 LED를 조합하여 특정한 광조건을 자유롭게 모사할 수 있고, 알루미늄 합금으로 이루어져 있기 때문에 wall loss를 특징하기 쉬우며, 지표 조건뿐 아니라 대류권 및 성층권 구름의 조건에서 발생하는 물리화학적 대기현상을 모사할 수 있다는 것이 강점이다. 이러한 특성을 이용하여 AIDAc 챔버에서는 에어로졸의 점성이 ice nucleation에 미치는 영향 (Wagner et al., 2012), 권운의 얼음형성과 결정 특성 (Schneider et al., 2021; Schnaiter et al., 2016; Hiranuma et al., 2014) 등 구름화학 연구 (Wagner et al., 2006) 등이 수행되었다. 광물 입자 (DeMott et al., 2015), 박테리아 (Amato et al., 2015), 토양먼지 (Steinke et al., 2016), 석탄비산재 (Umo et al., 2019), 식물 유래 유기물 (Steinke et al., 2020) 에어로졸의 ice nucleation activity를 관찰하고, 넓은 온도범위 (213~313 K)에서 α-pinene 및 β-caryophyllene 산화에 의한 SOAs 형성과 SOAs의 물리화학 특성 (Gao et al., 2023, 2022; Huang et al., 2018) 등 다른 챔버에서 구현하기 어려운 대기화학실험을 수행하고 있다.
SAPHIR
독일 Forschungszentrum Jülich GmbH (율리히연구소)의 SAPHIR (Simulation of Atmospheric PHotochemistry In a large Reaction Chamber) 챔버는 2008년 구축된 대형 옥외 챔버로 이중의 FEP 테플론으로 이루어진 270 m3의 수평 원통형 챔버이다 (Karl et al., 2004). SAPHIR 챔버는 DOAS (differential optical absorption spectroscopy), LIF 등의 광화학장비 및 CIMS-ToF, PTR-MS 등 다양한 질량분석기를 활용하여, OH, NO3, HO2 등의 라디칼과 NO2, OVOCs, HCHO 등 미량의 가스 분석기기에 대한 비교 검증 연구를 수행하였다 (Fuchs et al., 2012, 2010a, 2010b; Apel et al., 2008; Schlosser et al., 2007; Wyche et al., 2007).
2017년 BVOCs를 직접 주입하기 위한 식물 챔버인 SAPHIR+chamber를 구축하여 BVOCs로부터 형성되는 SOAs와 CCN activity를 연구하였으며 (Zhao et al., 2017; Dal Maso et al., 2016; Hohaus et al., 2016), CRDS (cavity ring-down spectroscopy), DOAS, open-path incoherent broadband cavity-enhanced absorption spectroscopy (IBBCEAS) 등의 첨단 광학장비를 구축하여 HONO, HCHO 검출기법에 대한 연구를 수행해왔다 (Glowania et al., 2021). 최근 연속형 흐름반응 실내 챔버인 SAPHIR* (star) chamber를 구축하였다.
일본
일본은 1970년 7월 18일 광화학 스모그가 관측되어 큰 피해를 입었다. 이에 따라 1979년 National Institute for Environmental Studies (NIES, 국립환경연구소) 챔버가 구축되었다. 이 챔버는 6 m3의 수평형 수직 실린더로, PFA로 코팅된 스텐레스스틸 (SUS 304)로 이루어져 있다. NIES 챔버는 최근까지도 SOAs의 volatility (Sato et al., 2019, 2018), VOCs의 OH-reactivity (Sakamoto et al., 2022; Sato et al., 2017), 오존생성 실험을 통해 O3 PR (ozone production regime)을 검증하는 등 (Morino et al., 2023) 다양한 연구를 활발히 진행하고 있다.
호주
호주는 CSIRO 챔버가 1983년 구축된 이후 (Nelson and Quigley, 1983) 지속적인 연구를 수행해왔다. 최초로 구축된 CSIRO 야외 챔버는 두 개의 20 m3 테플론백으로 구성되었으며, 2005년 18.1 m3의 CSIRO 실내 챔버가 구축되었다 (Hynes et al., 2005). 2015년부터는 24.76 m3 FEP 테플론백으로 이루어진 새로운 직사각형 실내 챔버를 구축하여 활용하고 있다 (White et al., 2018; Warren et al., 2008). CSIRO 챔버에서는 SAPRC-07, MCM 메커니즘을 평가하기 위한 실험을 활발히 수행해왔으며 (Li et al., 2020; White et al., 2018; Azzi et al., 2010; Hynes et al., 2005; Hess et al., 1992), 에탄올 함유 휘발유의 유증기 (Beer et al., 2011; White et al., 2010), 유칼립투스 잎에서 발생하는 1,8-cineole oxide (Iinuma et al., 2009), CO2 포집에 사용되는 2-amino-2-methyl-1-propanol (AMP) (Li et al., 2020)과 piperazine (White et al., 2015) 등 호주 대기환경과 탄소포집 기술에서 발생하는 화학물질의 대기환경 메커니즘과 영향을 연구해왔다.
영국
맨체스터 대학에는 18 m3 FEP 테플론백으로 이루어진 MAC (Manchester Aerosol Chamber)와 직경 1 m×높이 10 m의 스텐레스스틸로 구성된 구름 챔버인 MICC (Manchester Ice Cloud Chamber)가 있으며, 이는 스모그 실험과 구름화학 실험을 동시에 수행가능한 챔버이다 (Shao et al., 2022). MAC에서는 VOCs의 광화학반응으로 인한 SOAs의 생성 실험 (Alfarra et al., 2012)과 cloud condensation nuclei activity 실험 (Wang et al., 2022)이 진행되었으며, MAC와 MICC를 연동하여 ice nucleation 효과를 연구한 바 있다 (Frey et al., 2018).
중국은 중국과학원 (CAS, Chinese Academy of Sciences)과 중국환경과학원 (CRAES, Chinese Research Academy of Environmental Sciences), 베이징대학교 등에서 소형 반응기를 이용한 소규모 실험을 진행하여 국내외 저널에 발표하여 왔다 (Nie et al., 2002; Wu et al., 2001; Wang et al., 1989; Wang, 1998; Tang et al., 1982). 2000년대 중반 베이징을 비롯한 대도시를 중심으로 심각한 스모그 현상이 발생하였으며, 본격적인 스모그 챔버 연구에 대한 필요성이 대두되었다. 이에 따라 2000년대에 CAS와 칭화대학교 등에서 본격적으로 중형 챔버를 구축하였다. 중국은 최근까지도 지속적으로 새로운 타입의 스모그 챔버를 구축하고 있으며, 이를 통해 첨단의 대기화학 연구를 수행하고 있다.
CAS Chambers
CAS는 AIOFM-CAS (Hao et al., 2007), AIP-CAS (Jia et al., 2009; Du et al., 2007) 등의 소형 반응기를 구축하여 대기화학 연구를 수행해왔으며, 2014년 광저우 지화학연구소 (GIG)에 위치한 중국과학원 유기지화학연구소 (State Key Laboratory of Organic Geochemistry, GIG CAS)는 30 m3의 테플론 필름 실내 스모그 챔버인 GIG-CAS를 구축하였다 (Wang et al., 2014). CAS 생태과학연구센터 (RCEES-CAS)에는 0.1 m3 (Liang et al., 2011), 3 m3 (Song et al., 2018), 30 m3 (Chen et al., 2019a) 등 다양한 크기의 중소형 실내 스모그 챔버와 OFR (Liu et al., 2018)을 보유하고 있다. CAS에서 진행한 연구들은 중국 도시대기를 반영할 수 있도록 NOx 및 SO2 혼합 시스템에서 VOCs 광화학반응을 모사하였다 (Chen et al., 2019a; Liu et al., 2019; Deng et al., 2017; Liu et al., 2016).
칭화대 및 저장대
2007년 칭화대에서도 2 m3의 PFE 테플론 챔버를 구축하여 soot particle (Lu et al., 2008), (NH4)2SO4 (Lu et al., 2009), MnSO4과 ZnSO4 (Chu et al., 2013), FeSO4 (Chu et al., 2014)와 같은 다양한 seed 입자에서 광화학반응을 연구해왔다. 저장대학교의 CAPS-ZJU 챔버는 2015년 구축되어 2018년 보수된 챔버로 3 m3의 FPE 테플론 챔버이며, 챔버의 설치와 운영은 주로 CSIRO 챔버의 사례를 따랐다 (Li et al., 2020). CAPS-ZJU 챔버에서는 주로 NOx-SO2-VOCs (Li et al., 2017), NOx-NH4-VOCs (Chen et al., 2020) 시스템 등 석탄연소-광화학반응이 복합적으로 작용하는 시스템에서 대기 중 광화학반응을 연구하였다.
기타
CRAES의 주도로 구축된 CRAES Chamber는 56 m3의 FEP 테플론 필름백으로 만들어진 챔버로, 중국 최초의 옥외 챔버이다 (Li et al., 2021a). CRAES 챔버는 오존과 SOAs 형성과정에 관여하는 환경 요인 (Zhang et al., 2023)과 VOCs 혼합물의 영향 연구 (Li et al., 2021a)를 시작으로 활발한 연구를 수행하고 있다.
2022년 베이징화공대학에 석영으로 만들어진 최초의 챔버인 10 m3 크기의 중형 실내 챔버인 AHL/BUCT 챔버가 구축되었다 (Ma et al., 2022). 이 챔버는 wall effect를 제거하기 위해 스텐레스스틸 프레임에 5 mm 두께의 석영유리로 이루어져 있다. 이 챔버는 toluene-NOx 시스템과 α-pinene-ozone 시스템에서 박스모델과 부합되는 결과를 보여주었으며, 앞으로 SOAs 및 O3 생성반응에 활용할 계획이다.
2. 2 국내 스모그 챔버 연구동황
국내에서 서울시립대학교가 국내 최초로 2002년에 150 liter 부피의 테플론 반응백 2개와 blacklight 10개를 사용하여 실내 소형 스모그 챔버를 제작하여 실제 대기 중 가스상물질의 광화학반응 메커니즘 연구를 수행하였다 (Kim, 2002).
실제 대기질 실측을 통해 국내 스모그 현상을 연구해오던 KIST에서 2000년 초에 국가지정연구실 사업 지원을 통해 반응백 부피가 약 2.5 또는 6 m3인 실내 소형 쌍둥이 스모그 챔버를 제작하여 스모그 챔버 특성화를 수행하면서 (Lee et al., 2013, 2004; Bae et al., 2003), 실제 대기 및 synthetic mixture의 광화학반응 현상을 연구한 결과, 광도가 높을수록 실제 대기 및 toluene/NOx 혼합물의 오존 생성률이 증가하였고 (Lee et al., 2010), 광도가 높고 온도가 낮을수록 toluene/NOx 혼합물에 의한 이차 유기 에어로졸의 생성이 증가하였다 (Ju et al., 2007; Choi et al., 2006). 또한, 실제 대기를 이용하여 상대습도의 증가에 따른 입자 성장 현상을 관찰하였고 (Lee et al., 2006a; Kim et al., 2004), 실제 대기에 경유자동차 배기가스가 추가되면 오존 및 입자 생성이 감소하는 경향을 관찰함으로써, 서울시 대기 조건이 VOCs-limited 영역에 해당할 수 있음을 보였다 (Lee et al., 2006b).
국립환경과학원에서는 부피가 800 L인 테플론 반응백과 blacklight 22개를 이용하여 VOCs 종류 및 VOCs/NOx 비율에 따른 오존 생성 실험을 수행한 결과, 오존 생성 기여율은 ethylene, isoprene, toluene 등이 높았다 (Hong et al., 2003). 또한, VOCs/NOx 비율이 5, 7 ppbC/ppb일 때보다 3 ppbC/ppb일 때 오존 생성이 가장 적었다. 수도권 측정소의 VOCs/NOx 비율이 2.0~4.3 ppbC/ppb 범위이므로 수도권 대기는 VOCs-limited 조건인 것으로 판단하였으며, 도로이동오염원보다 유기용제 사용에 의한 배출량을 줄이는 것이 오존 저감에 효과적이라고 보고하였다 (Hong et al., 2003).
경북대학교에서 2009년경부터 부피가 7~8 m3인 실내 소형 챔버를 구축하여 오존 및 광화학반응에 의한 이차 유기 에어로졸 연구를 시작하였다 (Babar et al., 2016). 주요 전구물질로 대표적인 자연적 VOCs인 α-pinene과 인위적 VOCs인 toluene을 대상으로 NH3 등의 무기인자에 의한 비균일 미세먼지 생성을 주로 연구하였고, flow reactor 및 thermodenuder 등을 활용하여 이차 생성 유기 에어로졸의 연구를 심도있게 수행하였다 (Park et al., 2017; Babar et al., 2016).
국내에서 다시 스모그 챔버 연구가 활발하게 시작된 것은 미세먼지 범부처 프로젝트의 지원으로 수행된 “스모그 챔버를 이용한 동북아 미세먼지 생성기작 규명 (2017~2020)” 과제가 계기가 되었다. KIST는 고농도 미세먼지 사례에 미치는 무기염 성분 wet particle의 영향이 크다는 가설을 세우고 (Seo et al., 2020), 기존의 실내 소형 챔버를 이용하여 동북아 대기 조건인 high NOx 조건에서 VOCs별 다상반응에 의한 이차 입자 생성 실험을 수행하는 한편, 장거리 영향을 평가하는 데 필요한 27 m3 부피의 -10~40°C 범위 온도조절이 가능한 중형 스모그 챔버를 구축하고 성능을 평가하였다. 경북대에서는 toluene, m-xylene, ethylbenzene, α-pinene, isoprene 등의 주요 VOCs에 대해 SO2와 NH3가 포함된 다습한 (상대습도 ~60%) 동북아 대기 조건의 챔버 실험을 통해 two-product 모수를 도출하였고, 3차원 대기확산모델링인 CMAQ의 입력자료로 제공되어 예측 정확도 향상에 기여하였다.
미세먼지 범부처 프로젝트의 후속사업인 동북아-지역 연계 초미세먼지 대응 기술개발 사업단의 지원으로 수행하고 있는 “다중스케일 챔버를 활용한 초미세먼지 생성기작 규명 연구”를 통해 KIST에서는 국내정체, 국외유입, 국외유입 후 국내정체 등에 의해 발생하는 것으로 보고되고 있는 미세먼지 고농도 사례를 실험적으로 모사하기 위해 중형 스모그 챔버를 활용하여 다중일반응에 의한 생성 기작 규명 연구를 수행 중에 있다. 또한, 경북대에서는 benzene, 1,2,4-TMB, styrene, n-dodecane, cyclododecane에 대한 챔버 실험을 통해 two-product 모수화값 업데이트의 완성도를 높이고 있을 뿐만 아니라, aerosol liquid water content, aerosol pH, aerosol phase 등을 실시간으로 측정할 수 있는 기법 및 장치 등을 개발하여 입자 특성화 및 생성기작 규명에 활용하고 있다.
한편, 실제 대기와 태양광을 이용한 광화학반응 실험을 위해 ethylene tetra fluoro ethylene (ETFE) 재질로 1,163 m3 (길이 22 m, 폭 12 m, 높이 6 m) 부피의 반구형태인 대형 실외 스모그 챔버가 2021년 한국건설기술연구원 주관으로 경기도 연천군 옥산리에 구축되었고, SOAs 생성 연구뿐만 아니라 차량도로 개선용 TiO2 효율 평가, 공기청정기에 대한 미세먼지 저감 평가, 미세먼지 저감을 위한 버스정류장 수직녹화시스템 성능 평가 등에 활용되고 있다 (Kim et al., 2021; Song et al., 2021).
3. 연구 주제별 동향
3. 1 VOCs 산화 기작 연구
많은 VOCs precursor 중 toluene의 경우 대기 중에서 비교적 높은 농도로 방출되며, 높은 oxidation reactivity (9.7×10-12 cm3 mol-1 s-1 at 298 K)를 지니며 SOA yield가 크기 때문에 많이 연구되는 물질 중 하나이다. 보통 toluene 챔버 실험의 경우 OH 라디칼을 사용하는데, water vapor나 오존 UV photoloysis를 통해 OH 라디칼을 발생시킨다. 그림 3에서와 같이 OH 라디칼에 의해서 1차 산화된 물질 중 90%는 OH가 addition된 C7H9O2·가 되고 나머지 10%는 H가 제거된 형태인 C7H7·이 된다. C7H9O2·는 O2에 의한 추가적인 반응에 의해 수소가 제거된 형태를 거쳐 cresol을 생성하거나 O2 addition을 통해 benzylperoxy 라디칼을 생성한 후 분해되어 glyoxal, methylglyoxal과 같은 dicarbonyl을 생성하는 경로가 많이 연구되어 있다 (Ji et al., 2017). 최근 toluene SOAs 생성 연구에서는 SOAs 생성에 기여하는 무기인자들을 규명하는 연구나 중간생성체인 라디칼 (RO2·, RO· 등)에 관련된 kinetics 연구나 산화과정에서 NOx, NH3의 영향에 관한 연구들이 발표되고 있다.
중간생성체인 RO2· 라디칼은 VOCs 전구체의 자가산화 (autoxidation)를 통해 highly oxidized molecules (HOM, 보통 6개 이상의 산소원자를 가지는 자가산화를 통해서 가스상에서 보통의 조건에서 생성될 수 있는 물질을 의미함)을 생성하여 SOAs 생성에 기여한다 (Bianchi et al., 2019). 앞에서 설명한 toluene 산화과정처럼 RO2· 라디칼은 유기분자에서 수소원자가 떨어져 R· 라디칼을 생성한 후 산소와 반응하여 만들어진다. 그 후 RO2는 다양한 반응을 일으킬 수 있는데, inter 혹은 intramolecule 수소 제거반응을 통해 hydroperoxide 작용기와 alkyl 라디칼을 생성하여 산소분자에 의해 더 산화된 형태의 라디칼을 만들거나 분해되어 작은 라디칼을 발생시키게 된다. 초기에는 대기 중의 낮은 농도와 낮은 H-shift 가능성으로 주목받지 못했으나, 최근 연구에 의하면 극저 휘발성을 가지는 유기화합물이 이러한 자가산화과정을 통해서 생성된다는 것을 관찰하였다 (Ehn et al., 2014, 2012). 보통 방향족화합물에서 생성된 RO2의 자가산화반응 (autoxidation)의 HOM yield가 크고 O/C ratio가 1.09로 높게 나타났으며, 최근 보고에 의하면 방향족 화합물의 자가산화과정은 terpene의 자가산화과정과 유사하게 산화도가 높은 형태로 진행된다는 것이 보고되었다. 방향족 화합물의 자가산화 라디칼 체인 반응은 peroxy 라디칼에 의한 산성 C-H 결합 내 수소원자를 제거하거나 peroxy 기능성기를 형성하고 주변의 산소 분자와 여러 차례 반응을 반복하며, 특히 방향족 화합물의 경우 방향족 고리의 이중결합을 peroxy 그룹이 공격하여 산소다리를 형성하는 자가산화 형태로 발생하여 산소의 비율이 높아질 수 있다 (Molteni et al., 2018).
한편, 최근에 보고되고 있는 챔버 연구 내용은 실제 대기와 유사한 mixed VOCs precursor를 이용하여 진행한 실험들이 있는데, 인위적 VOC 혼합물을 사용하거나 인위적 VOCs와 식생 VOCs를 혼합하여 진행하기도 한다. 특히, 기존 single VOC 광화학반응에서 관찰된 결과와 상반되는 결과나 시너지 있는 결과를 통해 SOAs 생성 기작을 규명하고, 기존 SOAs 생성 기작을 보강하여 SOAs 생성 예측의 정확도를 높이고자 하였다. 최근 보고된 mixed VOCs precursor를 이용한 연구의 예를 아래에 간단히 소개하였다 (Li et al., 2022, 2021a).
Single VOC 실험 결과와 상반된 결과의 예로, 최근 중국에서 CAPS-ZJU (Complex Air Study-Zhejiang University) 챔버를 이용하여 식생 VOCs인 isoprene이 인위적 VOCs인 toluene, p-xylene과 혼합되어 있는 경우 isoprene의 산화과정이 인위적 VOCs의 SOAs 생성을 억제한다고 발표하였다 (Li et al., 2022). 본 실험에서는 100~150 ppb의 single VOC 및 mixed VOCs toluene+isoprene, p-xylene+isoprene 등 8가지 세트로 실험을 구성하여 진행하였으며, 모두 NOx가 있는 조건에서 SOAs 생성 및 gas phase chemistry를 관찰하였다. 그 결과 mixed VOCs의 조건에서 6시간 이상 광반응을 진행한 결과 particle 개수와 aerosol mass loading이 모두 80%까지 감소하였으며, SOAs의 화학조성 및 산화 정도가 역시 single VOC와 달라지는 것을 확인하였다. 또한 propene 실험과 비교하였을 때 isoprene으로 인해 aerosol nucleation 첫 생성 시작 시간이 늦춰진다는 것을 알 수 있었는데, 이는 isoprene이 new particle formation을 방해하기 때문으로 나타났다. 이를 규명하기 위해 몇 가지 모델 (SAPRC, CB6, MCM)을 이용하여 SOAs 생성 simulation을 진행하였는데, isoprene이 첨가된 후 toluene과 p-xylene의 광화학반응 정도가 줄어들었으며, 이는 alkene종과 같은 highly reactive 한 물질에 의해 OH 가 줄어드는 OH scavenging effect이다.
한편, 시너지 효과가 나타난 연구에서는 인위적 VOCs들이 혼합되어 있는 경우 개별 VOC에 의한 반응보다 더 많은 SOAs가 생성될 수 있다고 보고하였다 (Li et al., 2021a). Li 그룹은 n-dodecane과 1,3,5-trimethylbenzene이 혼합되어 있는 VOCs의 경우 개별 VOC가 산화과정을 통해 생성하는 SOAs보다 더 많은 SOAs가 발생된다는 것을 보고하였다. 본 연구에 의하면 자동차 배기가스에서 발생될 수 있는 두 가지 전구체 VOCs를 이용하여 챔버 실험을 진행하였고, 이 두 가지 물질에서 발생되는 중간생성물이 또다른 SOAs 반응 경로를 만들어 더 많은 SOAs를 생성한다는 것을 밝혀내었다. 이는 n-dodecane과 1,3,5-trimethylbenzene의 gas phase product로 carbonyl, hydroxyl, alcohol 물질들이 생성되는데, 이렇게 생성된 물질들이 particle phase에서 높은 aldehyde, ketone, alcohol류의 농도로 인해 acetal reaction이나 esterification reaction을 더 많이 거치게 되면서 SOA yield를 높이게 되는 것으로 추정된다. 따라서 이와 같이 혼합 VOCs 시스템의 챔버 실험 결과는 궁극적으로 수치모델링에서 과소평가되었던 SOAs 농도를 높이는 데 기여할 수 있다.
3. 2 이차 생성 입자의 화학조성 연구
챔버 실험에서는 일반적인 대기 중 OH 농도를 주입 혹은 생성하여 수 시간 동안 실험에 의해 생성된 입자의 O : C 비는 약 0.3 정도로 semi-volatile oxygenated organic aerosol (SV-OOA) 범위를 나타내기도 한다. 대기 중 일평균 OH 라디칼의 농도가 약 1.5×106 molecules/cm3 (25°C, 1기압에서 약 6.1×10-5 ppb)로 알려져 있으므로 (Mao et al., 2009), 24시간 노출 시간을 곱하면 1-day equivalent OH exposure는 1.3×1011 molecules·sec/cm3이다. 반면에 흐름반응기와 같은 실험에서는 더 높은 OH 노출을 통한 반응이 이루어지며, 이 결과로 O : C 비가 최대 1 정도로 크게 산화된 에어로졸 (low-volatile oxygenated organic aerosol (LV-OOA) 범위)을 생성하기도 하는데, 챔버 실험을 통해 SOAs의 생성과 노화를 실시간으로 분석하기 위해 HR-AMS와 같은 online 분석장비가 사용되어 왔다.
AMS 데이터 해석에서 OA (Organic Aerosol)의 변화는 OOA 스펙트럼에서 가장 주요한 산소 함유 이온인 m/z 44 (대부분의 경우 대기 자료에서 CO2+)와 m/z 43의 변화 강도에 따라 특성화할 수 있다. m/z 43 이온은 주로 OOA 구성요소의 C2H3O+ 및 HOA 구성요소의 C3H7+이다. OOA에서 m/z 44 이온은 대부분 산 (acid) (Duplissy et al., 2011) 또는 에스테르 (esters)와 같은 산으로부터 기원된 물질에 기인한다고 알려져 있는 반면, m/z 43 (C2H3O+) 이온은 주로 비산성 산화물 (non-acid oxygenates)로 인한 것으로 알려져 있다. 산성 및 비산성 산화물은 모두 대기 중 OA에서 관찰된다고 알려져 있다 (Russell et al., 2011; Decesari et al., 2007). AMS fragments 중 f44 (구성 요소 스펙트럼의 m/z 44와 총 신호 비율)와 f43 (구성 요소 스펙트럼의 m/z 43과 총 신호 비율)을 그래프로 그린 삼각형 플롯 (Triangle plot: f44 vs. f43)에서 HOA, SV-OOA, LV-OOA로 산화가 진행될수록 삼각형의 하단에서 꼭지점을 향해 이동하는 경향이 있으며 (Liu et al., 2018), VOCs의 종류와 반응 라디칼 종류에 따라서도 차이를 보이는 것으로 알려져 있다 (Docherty et al., 2018).
Heald et al. (2010)은 Van Krevelen 다이어그램 (H : C vs. O : C) (Krevelen and Baans, 1950)을 사용하여 전체 OA 구성 변화를 특성화한 바 있다. 최근 보고된 HR-AMS 관측 및 챔버 연구 결과에서는 Van Krevelen 다이어그램에 좁은 범위를 차지하는 것으로 나타났고, 그림 5에서와 같이 대부분의 데이터 기울기가 ~-1 인 직선에 따라 군집되어 있으며, 이는 카보닐 및 알코올 부분이 동시에 증가함에 따라 발생한 것임을 나타낸다.
3. 3 입자 생성 인자 영향 연구
스모그 챔버 실험에서 이차 미세먼지의 생성에 영향을 미치는 주요 인자는 VOCs의 종류 및 초기 농도, VOCs/NOx 농도비, 산화제 종류 및 농도, 추가된 무기가스의 종류 및 농도, seed 입자의 산성도, 기상요소 (기온, 상대습도, 광원의 종류 및 세기 등) 등 매우 다양하다. 이차 유기 에어로졸 생성률 (SOA yield)은 인위적 개별 VOC의 광화학반응에 의해 생성된 입자의 질량농도를 반응에 의해 소모된 VOCs의 질량농도로 나눈 무차원수로 정의한다. 이 값이 SOAs 생성 특성의 지표로 널리 활용되고 있을 뿐만 아니라, 대기확산모델링에서 SOAs 생성 농도를 모사하기 위해 이용되므로, 본 연구에서는 다양한 인자의 입자 생성 영향을 평가하는 데 SOA yield를 사용하였다. 최근까지 보고된 인위적 VOCs의 SOA yield가 Srivastava et al. (2022)에 정리되어 있다. 하지만, 동일 VOCs라도 VOCs/NOx 농도비에 의한 영향뿐만 아니라, gas-particle partitioning에 의해 유기 에어로졸의 농도에 의한 영향도 받기 때문에, yield 값 자체만 단순비교해서는 안된다. NOx 농도가 30 ppb 이상, VOCs/NOx> 10ppbC/ppb인 조건에서 유기 에어로졸의 농도 27 µg/m3이라고 가정한 경우 개별 VOC별 SOA yield를 Gu et al. (2021)에서 비교할 수 있다. CMAQ v5.1의 AERO6 모듈에서 적용하고 있는 two product 모수화 값을 이용하여 주요 VOCs별 SOA yield 곡선을 그림 6에 나타내었다.
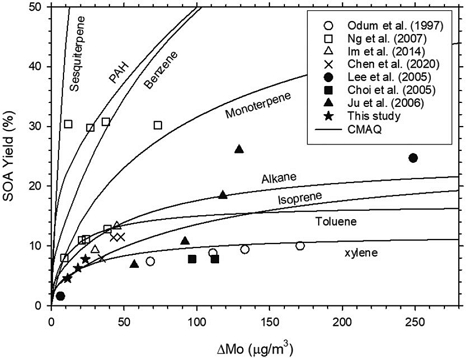
SOA yield versus organic aerosol mass. Data from Lee et al. (2005), Choi et al. (2005), and Ju et al. (2006) and this study were re-calculated with SOAs density of 1.24 g/cm3 (Ng et al., 2007), and data from Lee et al. (2005) was wall-loss corrected.
동일 VOCs의 경우에도 VOCs/NOx 농도비에 따라 SOA yield가 변하는 것으로 보고되었는데, VOCs/NOx 비율이 증가할수록 SOA yield가 증가하다가 최고값을 나타낸 후 감소한다는 연구 결과가 다수 보고되었다. SOA yield가 최고값을 나타내는 임계 VOCs/NOx 농도비는 VOCs 종류에 따라 다르게 나타났는데, isoprene (C5H8)의 경우 ~2 ppbC/ppb (Kroll et al., 2006), camphene (C10H16)의 경우 ~5 ppbC/ppb (Li et al., 2022), β-pinene (C10H16)의 경우 ~12 ppbC/ppb (Sarrafzadeh et al., 2016), 1,3,5-TMB의 경우 ~14 ppC/ppb (Yang et al., 2020), ally acetate (C5H8O2)의 경우 ~14 ppbC/ppb이었다 (Wang et al., 2021). 여러 연구자의 실험 결과를 종합적으로 분석해보면, toluene 의 경우 ~16 ppbC/ppb에서 SOA yield가 최대값이었다 (Chen et al., 2020, 2019a; Ng et al., 2007). 한편, 4~5 ppb 수준의 longifolene (C15H24), aromadendrene (C15H24)의 실험에서 NOx 농도가 증가할수록 SOA yield가 증가하였던 결과는 매우 낮은 VOCs 농도 (4~5 ppb) 및 매우 낮은 VOCs/NOx 비율 (0.13~0.74 ppbC/ppb) 조건에서 3~5 ppm의 H2O2 (H2O2/VOCs 농도비>600 ppm/ppm)에 의한 OH 생성 영향 때문일 것으로 추정된다 (Ng et al., 2007).
한국의 경우 겨울철 미세먼지의 고농도 사례 발생이 빈번하므로, 기상 요소 중 기온의 영향에 관심이 많은데, 온도가 SOA yield에 미치는 영향은 논란의 여지없이 모든 연구들이 동일한 경향을 나타내었다. 즉, 온도가 낮을수록 SOA yield가 증가하였는데, 이는 온도가 낮을수록 가스상 반응속도는 낮아지지 만, 그 영향보다 SOAs의 포화증기압이 낮아져서 gas-to-particle partitioning이 더 활발하기 때문이며, SOAs별 상평형 계수 K를 결정짓는 휘발엔탈피 물성치로 설명될 수 있다. 유기 에어로졸의 농도 (Mo)에 따라 SOA yield는 변하므로, Mo=10 µg/m3 조건에서 온도가 20°C 낮을 때, 즉, 상온 (20°C) 대비 초저온 (0°C) 또는 고온 (30°C) 대비 저온 (10°C)의 SOA yield 비율 (YT-20/YT)은 BVOCs의 경우 limonene (1.5~2.3), α-pinene (1.4~2.2), β-pinene (1.7~2.7)이었고 (Saathoff et al., 2009; Svendby et al., 2008), AVOCs의 경우 n-undecane (1.8), toluene (1.3~2.1), m-xylene (1.6~3.4) 등으로 보고되었다 (Svendby et al., 2008; Takekawa et al., 2003).
동북아 대기조건의 특징인 다습한 조건에서 이차 미세먼지 생성이 증가할 수 있다. (NH4)2SO4 혹은 NaCl과 같은 조해성 seed 입자가 있는 경우 RH가 낮을 때보다 높을 때 toluene SOA yield가 증가하였는데, acid catalysis 반응 또는 glyoxal과 같은 수용성 물질의 aqueous reaction으로 설명되었다 (Jia and Xu, 2018; Liu et al., 2018; Faust et al., 2017; Kamens et al., 2011). 반면, 중성 또는 산성 seed 입자가 있어도 RH ~50%일 때 RH ~20%에 비해 SOA yield가 감소한 연구도 있었는데, H2O2/toluene 비율이 약 4~6 ppm/ppm인 low-NOx 조건이었다 (Cao and Jang, 2010). Seed 입자가 없는 경우 SOAs의 hygroscopic growth를 고려한 결과, RH가 증가할수록 초기 농도 60~80 ppb인 toluene 및 m-xylene의 SOA yield가 증가하다가 임계 RH 50%에서 최고값 (RH 2% 대비 약 2배 수준)에 도달한 후 다시 감소하지만, RH 90%에서 RH 2% 대비 약 1.04~1.12배 높았는데, 이는 RH에 따른 가스상 OH 라디칼의 농도 증감 때문이라고 설명하였다 (Chen et al., 2021). VOCs의 농도 감소 추세를 이용하여 추정한 OH 농도는 상대습도가 증가할수록 증가하다가 RH 50%에서 최고를 나타낸 후 다시 낮아지는 경향을 나타냈는데, 이는 입자 또는 반응백 벽면에 흡수되어 감소되기 때문으로 추정하였다 (Chen et al., 2021). 초기 농도가 약 1 ppm 수준인 toluene의 경우 RH ~80% 조건의 SOA yield가 RH ~10%보다 약 1.6배 높았다 (Jia and Xu, 2018). Isoprene의 경우 RH ~80% 조건의 SOA yield가 RH 약 10%보다 약 1/3 수준으로 낮았는데, 이는 고습도 조건에서 oligomerization reaction이 억제되었기 때문으로 보고되었다 (Jia and Xu, 2018). 이와 달리, RH 5~75% 범위에서 RH가 증가할수록 p-xylene의 SOA yield가 증가하는 경향이었다고 보고되었는데 (Healy et al., 2009), 이 경우 SOAs의 hygroscopic growth를 고려하지 못했기 때문에 고습도 조건에서 aerosol water content까지 SOAs로 간주되었을 가능성이 있다. 반면, seed 입자가 없는 경우 RH가 증가할수록 toluene 또는 m-xylene의 SOA yield가 감소하는 경향을 보고한 연구 결과들도 있는데, 이때 H2O2/VOCs 비율이 2~42 ppm/ppm으로 높았던 차이점이 있었다 (Zhang et al., 2019). 이러한 H2O2/VOCs 비율의 영향을 분석한 연구에서 cyclohexene SOA yield가 건조조건 (RH 13~16%)보다 다습조건 (RH ~70%)에서 높은 이유 (H2O2/VOCs 0.4~0.8 ppm/ppm 조건)와 낮은 이유 (H2O2/VOCs 1.3 ppm/ppm 조건)의 주요 원인이 H2O2/VOCs 비율의 차이 때문이라고 설명하였다 (Liu et al., 2019). 요약하면, 건조조건에서는 OH 라디칼의 농도가 상대적으로 낮은 반면, 고습조건에서는 OH 라디칼의 생성이 많아도 aerosol 및 chamber wall에 흡수되어 농도가 낮아질 뿐만 아니라 입자의 oligomerization 반응이 억제되므로, 중간 습도인 RH 50%에서 SOA yield가 최고를 나타내었다. 하지만, OH 라디칼을 생성시키는 산화제를 주입한 경우 SOA yield는 RH의 영향과 함께 산화제/VOCs 비율의 영향도 받는 것으로 추정된다.
실내 스모그 챔버에서 사용하는 인공광원의 경우 광원의 종류 및 광도의 영향에 대해서도 연구되었는데, 350 nm blacklight (NEC FL40SBL), blue blacklight (Sylvania F36W BLB), argon arc lamp에 따른 m-xylene, SOA yield 차이는 Mo 농도 20 µg/m3 이하 에서 크지 않았고, 광도가 클수록 SOA yield가 증가하였다 (White et al., 2018; Warren et al., 2008).
3. 4 스모그 챔버 이용 유해성 연구
스모그 챔버는 LA 스모그 등 유해 대기현상을 규명하기 위해 촉발되었기 때문에, 스모그 및 대기화학반응 생성물의 유해성을 규명하기 위해 다양한 연구를 수행해왔다. 1985년부터 UNC와 EPA의 주도로, 스모그 챔버에서 발생한 입자와 가스를 균주에 직접 노출하여 돌연변이를 관찰하는 연구를 수행하였으며 (Shepson et al., 1987, 1985; Kleindienst et al., 1986; Kamens et al., 1984), 이를 통해 O3/NO2 등에 의한 VOCs의 노화과정과 분자의 염소원자가 돌연변이성을 증가시키는 데에 큰 영향을 끼친다는 사실을 발견하였다. 이후로도 스모그 챔버 생성 에어로졸을 폐 세포 등에 노출시키는 in-vitro 실험 (Chowdhury et al., 2018), 쥐 등 동물에 노출시키는 in-vivo 실험 (McGee Hargrove et al., 2018)이 수행되어 왔다.
미세먼지가 독성을 나타내는 첫 번째 원인은 미세먼지에 함유된 극미량 오염물질이 발현하는 독성이다. 중금속, 다이옥신 등 극미량 유해물질은 무기염 및 기타 탄화수소에 비해 농도는 낮더라도 매우 높은 유해도를 보인다. 스모그 챔버에서는 다환방향족탄화수소 (PAHs) (Zhang et al., 2022), 잔류성유기화합물 (POPs) (Vera et al., 2015; Lutes et al., 1992), 과불화화합물 (Ren et al., 2019), 농약 (Muñoz et al., 2018; White et al., 2015), 조류독소 (Sem et al., 2022; Jang et al., 2020), 유기실록산 (Latimer et al., 1998) 등 유해화학물질의 대기화학반응 연구를 통해 구조변화와 이에 따른 유해성 판단의 기초가 될 수 있는 자료를 생성해왔다. 특히 이들의 대기 중 산화과정은 유기성분의 입자상 분배를 증가시키고, 입자에서 shielding effect 로 인해 장거리 이동성과 이에 따른 노출농도 기간을 증가시키는 역할을 한다 (Zhou et al., 2019; Mu et al., 2018; Socorro et al., 2017). 뿐만 아니라 대기 중 질산화 및 산화과정 (Riva et al., 2015; Zimmermann et al., 2013)에 따라 독성이 증가하기도 한다 (Clergé et al., 2019; Bandowe and Meusel, 2017).
미세먼지가 독성을 일으키는 두 번째 경로는 미세먼지가 일으키는 산화스트레스이다 (Bates et al., 2019; Crobeddu et al., 2017). 미세먼지의 독성 기작은 대부분 산화스트레스와 염증반응에 달려있으며 (Arashiro et al., 2018), 최근 미세먼지가 산화스트레스를 발생시킬 수 있는 OP (oxidative potential)가 미세먼지의 유해성을 대표할 수 있는 지표로 제안되어 사용되고 있다 (Zhang et al., 2022; Gao et al., 2020; Bates et al., 2019; Lavigne et al., 2018; Shiraiwa et al., 2017). 미세 먼지의 조성에 따라 달라지는 OP는 총 농도보다 유병률/조기사망률을 효과적으로 예측하는 것으로 평가받고 있다 (Rule and Koehler, 2022; Shiraiwa et al., 2017; Lakey et al., 2016; Weichenthal et al., 2016; Steenhof et al., 2011). 대기 중 미세먼지에서 OP를 발생할 수 있는 기작과 요인은 여전히 많은 연구가 필요한 상태이며, 스모그 챔버를 이용하여 물질과 조건에 따라 SOAs의 OP를 측정하고 영향인자를 밝히는 연구가 수행되어 왔다. OP는 미세먼지의 기원, 반응 VOCs와 유기생성물, 중금속, 나노입자의 유뮤, 대기 중 화학반응에 따른 노화 여부와 조건에 따라 큰 차이를 보였다 (Arashiro et al., 2018; Chowdhury et al., 2018; Park et al., 2018; Jiang et al., 2017, 2016; Tuet et al., 2017a, 2017b; Kramer et al., 2016; Rattanavaraha et al., 2011).
미세먼지 중 OP를 측정하기 위해서는 ascorbic acid (AA), glutathione (GSH), cysteine (CSH), dithiothreitol (DTT) 등의 항산화제를 사용하거나, 전자자기공명 (Electroparamagnetic Resonance)을 이용해 라디칼을 측정한다 (Shahpoury et al., 2022, 2019; Bates et al., 2019; Park et al., 2018; Crobeddu et al., 2017; Fang et al., 2016). 각 측정법은 연구마다 각기 다른 결과를 내며, 일부 연구에서는 생체 내에서 ROS 상관성이 떨어지는 경우가 보고되기도 한다 (Øvrevik, 2019). 이러한 한계를 극복하기 위해 입자의 크기와 성분, 호흡기 침투 범위의 메커니즘에 대한 더욱 정밀한 고려가 필요하며 (Dovrou et al., 2023; Fang et al., 2019), OP 분석법의 정밀화 및 표준화 (Jiang et al., 2019), 수명이 짧은 화학종을 손실 없이 측정하기 위해 실시간 측정법이 개발될 필요가 있다 (Zhang et al., 2022).
또한 미세먼지의 크기에 따라 호흡기 침투 깊이와 노출 부위가 달라지며, 이에 따라 폐액과 노출경로가 달라진다. 이를 극복하기 위해서는 입자 크기에 따라 정밀하게 OP를 측정하고, 추출 용매 (Pietrogrande et al., 2021; Fang et al., 2017)를 적절히 선정하여 미세먼지 구성 성분의 용출률과 OP, ROS 생성 메커니즘을 정밀하게 규명할 필요가 있다.
3. 5 구름응결핵 연구
대기 에어로졸 중 일부는 구름응결핵 (Cloud Condensation Nuclei, CCN)의 역할을 하여, 구름의 생성과 발달에 영향을 미치고 구름의 미세물리적 그리고 광학적 특성에 영향을 준다. 에어로졸 입자가 CCN으로 작용하는 능력인 CCN 활동성은 입자 크기와 화학적 조성에 따라 달라진다. 현재 구름응결핵 활동성 은 상용화 장비인 DMT사의 CCN-Counter (Lathem and Nenes, 2011; Rose et al., 2008; Roberts and Nenes, 2005)를 에어로졸 크기 분류 장치와 결합하여 측정하는 방법이 일반적이다. 측정된 구름응결핵 활동성은 다중 성분 혼합물의 수성 (water activity)을 특성화하기 위한 단일 흡습성 매개 변수인 κ로 표현할 수 있다 (Petters and Kreidenweis, 2007). 흡습성 매개 변수 κ값이 클수록 구름응결핵 활동성이 높음을 의미한다.
무기성분의 CCN 활동성은 널리 알려진 반면 유기 에어로졸은 물리적, 화학적 특성이 매우 복잡하며, 희석, 혼합 및 산화적 노화와 같은 대기과정을 거치면서 유기 에어로졸은 그 특성이 변화하며 CCN 활동성에도 영향을 미친다. 탄화수소와 산화 조건에 따른 이차유기 에어로졸의 CCN 활동성에 관한 다양한 연구들이 스모그 챔버 실험을 통해 이루어져 왔다.
이전의 많은 연구들은 세 가지 주요 monoterpene 성분인 α-pinene, β-pinene, limonene의 산화로부터 생성된 SOAs의 CCN 활동성에 집중하였다 (Wang et al., 2022; Fofie et al., 2018; Liu et al., 2018; Yuan et al., 2017; Zhao et al., 2016; Alfarra et al., 2013; Frosch et al., 2011; Asa-Awuku et al., 2009; Jurányi et al., 2009; King et al., 2009; Petters et al., 2009; Wex et al., 2009; Duplissy et al., 2008; Engelhart et al., 2008; King et al., 2007; Prenni et al., 2007; Varutbangkul et al., 2006; Huff Hartz et al., 2005; VanReken, 2005). King et al. (2009)에서는 (NH4)2SO4과 α-pinene 혼합입자의 κ값으로 0.12~0.16의 범위를 제시했으며, Frosch et al. (2011)은 α-pinene ozonolysis SOAs의 O/C 비율과 κ 간의 관계식 (κ=(0.071±0.02)×(O/C)+(0.0785±0.009))을 제시하였다. Huff Hartz et al. (2005)은 monoterpene 4종 (α-pinene, β-pinene, 3-carene, limonene)과 sesquiterpene 3종 (β-caryophyllene, α-humulene, α-cedrene)의 ozonolysis SOAs에 대해 실험한 결과 1% 과포화도에서 CCN 활동성은 monoterpene이 sesquiterpene보다 높았고, 0.3% 과포화도에서 CCN 활동성은 α-pinene, β-pinene 3-carene 그리고 limonene 순으로 높은 경향을 보였다. 최근에는 다양한 SOAs에 대한 CCN 활동성 실험 결과들이 제시되고 있으며, isoprene의 κ값 은 0.1~0.12 (Engelhart et al., 2011, 2008; Gunthe et al., 2009), γ-terpinene의 κ값은 0.23을 보였다 (Bouzidi et al., 2022). 이 외에도 Asa-Awuku et al. (2010)에서는 alkenes, monoterpenes, sesquiterpene parent hydrocarbon으로부터 생성된 수용성 SOAs의 κ값이 무기염과 거의 유사한 범위 (0.26~0.33)라고 보고하였다. Prenni et al. (2007)과 Engelhart et al. (2008)에서는 seed 입자가 없는 상태에서 α-pinene과 3-carene를 포함한 여러 타입의 ozonolysis SOAs와 광분해반응으로 생성된 toluene SOAs의 κ값을 측정한 결과 각각 0.1±0.04, 0.15±0.08이었다. Yuan et al. (2017)에서는 대기와 비슷한 조건에서 terpenes의 오존분해반응으로 생성된 SOAs의 κ값 (α-cedrene 0.12~0.24, α-humulene 0.06~0.16)을 제시하였다.
최근에는 PAM-OFR (Potential Aerosol Mass Oxidation Flow Reactor)를 이용한 SOAs의 CCN 활동성 연구가 이루어지고 있다. PAM-OFR은 대기 중 며칠 동안의 산화과정을 실시간으로 시뮬레이션하는 고도의 산화 환경을 만들어주는 장비이다. Massoli et al. (2010) 의 연구에 따르면 대기 노화의 0.5에서 10일에 해당하 는 산화 노출에 대해 세 가지 전구체 (α-pinene, 1,3,5-trimethylbenzene (TMB) and m-xylene)로부터 생성된 SOAs의 CCN 활동성을 특성화하였으며, CCN 활동성은 O/C 비율 0.38에서 0.98의 범위 내에서 SOAs의 κ값과 양의 상관관계를 관찰하였고, 실제 대기의 OOA 의 흡습 특성과 유사함을 확인하였다. Lambe et al. (2011)은 PAM-OFR 을 이용하여 n-decane, isoprene, α-pinene을 포함한 일곱 가지 VOCs 전구체로 생성된 SOAs 및 OPOA (Oxidized Primary Organic Aerosol)의 CCN 활동성을 측정하였다. O/C 비율이 0.05에서 1.42 범위 내에서 κ값은 0.008에서 0.28 값을 가졌으며, κ값과 O/C 비율 간의 관계는 선형 함수로 표현할 수 있었다 (κorg=(0.18±0.04)×O/C+0.03). 이러한 선형 관계식은 3차원 수송모델인 Community Multiscale Air Quality (CMAQ)에서 유기 에어로졸의 κ값 도출에 이용되었다 (Pye et al., 2017).
Suda et al. (2012)에서는 고성능 액체 크로마토그래피에 CCN-Counter를 결합하여 SOAs의 극성 분리 성분에 따라 κ값을 도출하는 새로운 방법을 제시하였다. 일련의 VOCs 및 산화제를 이용하여 총 24가지 실험을 통해 생성된 SOAs의 κ값은 0~0.4까지 넓게 분포하였다. Suda et al. (2014)에서는 동일한 방법으로 functional group의 영향을 조사한 결과, 그림 7과 같이 수산기 (hydroxyl) 또는 메틸렌기 (methylene group)의 경우 O/C 비율이 증가할 때 κ가 증가하는 경향이 강하게 나타났으며, Functional group당 κ의 증가는 hydroxyl의 경우 carboxyl과 hydroperoxide보다 상대적으로 컸으며, nitrate와 methylene의 경우 κ값이 증가하지 않고 감소하였다.
4. 요약 및 전망
스모그 챔버 연구는 시시각각 변하는 대기현상을 이해하기 위해 다양한 변수가 제어된 실험을 통해 현장 모니터링 연구에서 제시된 가설을 증명하기도 하고, 대기확산모델링의 모수값들을 업데이트하거나 새로운 반응/생성/변환 기작 등을 추가함으로써 수치모델링 모사를 개선하는 데 기여할 수 있는 매우 유용한 방법론이다. 이러한 스모그 챔버 연구 결과의 효율성 및 유용성을 유지하거나 극대화하기 위해서 고려하거나 개선해야 할 필요가 있는 이슈들을 몇 가지 제시하면서 향후 연구동향을 함께 전망하고자 한다.
첫째, 스모그 챔버의 반응백에 의한 배경반응성에 대한 고려가 필요하다. Izumi and Fukuyama (1990)에 따르면 background aerosol formation을 확인하기 위해 HC 없이 water vapor (RH 50%)와 NOx (10 ppb)의 혼합물로 Xe lamp를 켜서 광화학반응을 일으키는 blank test 결과 200분 후에 particle이 생성되어 최고 3~4 μm3/cm3의 부피 (밀도를 1 g/cm3로 가정하면 3~4 μg/m3)까지 생성되어서, HC를 대기농도 수준 (0.01 ppm 이하)으로 낮추지 못하고 1 ppm 수준으로 실험하였다고 보고하였다. 그동안 스모그 챔버 연구자들은 실제 대기환경 조건의 농도 수준으로 가능한 가깝게 실험하고자 노력해왔다. 대표적인 AVOCs인 toluene을 예로 들자면, 2006년까지 SOAs 생성 실험의 toluene 초기 농도는 대부분 1 ppm 이상이었다가 (최소농도는 0.5 ppm 수준), 2007년에 0.010~0.186 ppm의 저농도 실험 결과가 보고되기 시작하였으나 (Hu et al., 2007; Kroll et al., 2007; Ng et al., 2007), background aerosol formation의 영향에 의한 설명은 미흡하였으며, Leskinen et al. (2015)의 경우 초기 toluene 농도가 18~30 ppb이었는데, background aerosol formation은 약 1.9 μg/m3으로 약 11~34%를 차지하여 무시할 수 없는 수준이지만, 실험마다 변동이 크고 구분하기 어려워서 toluene SOA yield 계산에서 고려하지 못하였다. Toluene 초기 농도가 수십 ppb 수준으로 매우 낮은 저농도 실험에서 산화제를 추가하는 경우, 생성된 SOAs의 농도가 10 μg/m3 이상이 되어 background 오염 영향이 크지 않을 수 있지만 (Ng et al., 2007), 산화제 주입 없이 실험하여 SOAs 농도가 1.6 μg/m3으로 매우 낮은 경우 (Chen et al., 2017) background 오염 영향이 클 수 있으므로, 이를 고려한 결과 분석이 필요하다.
둘째, 주요 실험 인자들이 SOA yield에 미치는 영향을 분석하는 경우, 생성된 SOAs의 농도인 Mo를 고려할 필요가 있다. 즉, VOC/sNOx 비율의 영향을 예로 들면, 초기 VOCs 농도를 고정하고 NOx 농도를 변화시킨다든지, 반대로 NOx 농도를 고정한 채 VOCs 농도를 변화시키면서 챔버 실험을 하는 경우, 생성되는 Mo 농도가 변화하기 때문에 이로 인한 영향이 SOA yield에 포함되게 된다. 따라서, 오존 등농도 곡선과 유사하게, VOCs (ppbC)와 NOx (ppb)에 따른 Mo 등농도 곡선을 도출하는 것이 필요하다. 이러한 예를 Toluene, TMB, Xylene, Octane 혼합물의 광화학반응 1시간 후 입자의 등농도 곡선을 그린 Vivanco et al. (2011)에서 볼 수 있다. 다양한 VOCs, NOx 농도에 대한 실험 데이터가 있는 Yang et al. (2020)을 이용하여 그림 8과 같이 표현할 수 있다. 이 경우에도 VOCs/NOx 비율이 5 ppbC/ppb 이하인 실험 데이터가 존재하지 않는데, 추가로 산화제를 주입하는 경우 등농도 곡선의 경향이 달라질 가능성이 있다. 따라서, 실제 대기현상을 잘 모사할 수 있으면서 low-NOx 및 high-NOx 조건에서 일관된 Mo 경향성을 확보할 수 있는 실험 방법을 도출하거나, 적합한 box modeling 등을 이용하는 것이 유용할 것으로 생각된다.
셋째, 그동안 대부분의 연구에서 실험 결과 해석의 용이성을 위해 주간반응이나 야간반응을 별도로 실험하였으나, 향후에는 미세먼지 고농도 사례의 원인으로 추정되는 장기간 국내정체의 영향을 이해하기 위해 주간반응과 야간반응이 반복되는 다중일반응 실험이 필요하다고 판단된다. 초기에는 단일 VOC로 실험을 수행하였으나, 이후 혼합 VOCs로 실험함으로써 VOCs별로 생성된 product들 상호 간의 반응에 의한 영향을 분석하는 경향과 마찬가지이다. 실제 대기환경에서는 배출이나 수송의 영향을 배제하더라도 혼합고만의 증감에 따라 농도가 감소하거나 증가하는 것을 포함하여 스모그 챔버 실험에서 완벽하게 모사하기는 어렵더라도, 다중일반응을 통해 온도/상대습도/aerosol water content 등의 변화로 인한 비균질반응의 영향을 살펴볼 수 있을 것으로 기대된다.
넷째, 단일 오염 현상 연구보다 복합적인 오염 현상 연구가 필요하다. 예를 들어, 초기에는 오존 오염 연구도 활발하였으나 최근에 미세먼지 연구에 집중하면서 오존 관련 연구가 상대적으로 약화된 경향을 보인다. 그러나, 국내외적으로 미세먼지는 점차 개선되고 있으나 오존은 여전히 감소되지 않고 증가하고 있는 추세이므로, 미세먼지와 함께 오존과 유해물질 등의 생성과 변환과정을 함께 분석하는, 복합적인 오염 현상 규명 연구가 필요하다.
다섯째, 향후 대기환경/기후변화 융합 연구가 필요하다. 대기오염은 기후변화를 심화시키고, 기후변화는 다시 대기환경을 악화시키는 상호 영향을 주고받는 것으로 알려져 있으므로, 스모그 챔버와 구름 챔버를 함께 연동함으로써 가스-에어로졸-구름-기후변화간 상호 영향 연구를 종합적으로 수행할 수 있는 첨단 연구설비가 필요하다.
여섯째, 국내 관련 연구자들이 스모그 챔버 설비를 활용할 수 있는 공동연구 협업 체계의 도입이 필요하다. 공동연구를 통한 시너지 효과 및 원천연구 활성화를 도출하기 위해 유럽연합의 스모그 챔버 공동연구 플랫폼인 EUROCHAMP 2020과 유사하게 타 연구기관과 함께 공동연구를 수행할 수 있는 체계가 필요하다고 생각된다. 위와 같은 다양한 노력들을 통해 스모그 챔버 연구가 관련 환경 연구자들의 연구 교류와 소통의 허브가 될 수 있기를 기대하며, 효과적이고 과 학적인 환경 정책을 수립하는 데 기여할 수 있기를 바란다.
Acknowledgments
이 연구는 과학기술정보통신부의 재원으로 한국연구재단 - the Fine Particle Research Initiative in East Asia Considering National Differences (FRIEND) project (2020M3G1A1114556)와 KIST 대기환경복합대응사업 (2E32431)으로 지원되었기에 감사드립니다.
References
-
Ahmed, E., Kim, K.-H., Shon, Z.-H., Song, S.-K. (2015) Long-term trend of airborne particulate matter in Seoul, Korea from 2004 to 2013, Atmospheric Environment, 101, 125-133.
[https://doi.org/10.1016/j.atmosenv.2014.11.024]
-
Alfarra, M.R., Good, N., Wyche, K.P., Hamilton, J.F., Monks, P.S., Lewis, A.C., McFiggans, G. (2013) Water uptake is independent of the inferred composition of secondary aerosols derived from multiple biogenic VOCs, Atmospheric Chemistry and Physics, 13, 11769-11789.
[https://doi.org/10.5194/acp-13-11769-2013]
-
Alfarra, M.R., Hamilton, J.F., Wyche, K.P., Good, N., Ward, M.W., Carr, T., Barley, M.H., Monks, P.S., Jenkin, M.E., Lewis, A.C., McFiggans, G.B. (2012) The effect of photochemical ageing and initial precursor concentration on the composition and hygroscopic properties of β-caryophyllene secondary organic aerosol, Atmospheric Chemistry and Physics, 12, 6417-6436.
[https://doi.org/10.5194/acp-12-6417-2012]
-
Amato, P., Joly, M., Schaupp, C., Attard, E., Möhler, O., Morris, C.E., Brunet, Y., Delort, A.-M. (2015) Survival and ice nucleation activity of bacteria as aerosols in a cloud simulation chamber, Atmospheric Chemistry and Physics, 15, 6455-6465.
[https://doi.org/10.5194/acp-15-6455-2015]
-
Apel, E.C., Brauers, T., Koppmann, R., Bandowe, B., Bossmeyer, J., Holzke, C., Tillmann, R., Wahner, A., Wegener, R., Brunner, A., Jocher, M. (2008) Intercomparison of oxygenated volatile organic compound measurements at the SAPHIR atmosphere simulation chamber, Journal of Geophysical Research: Atmospheres, 113, D20307.
[https://doi.org/10.1029/2008JD009865]
-
Arashiro, M., Lin, Y.-H., Zhang, Z., Sexton, K.G., Gold, A., Jaspers, I., Fry, R.C., Surratt, J.D. (2018) Effect of secondary organic aerosol from isoprene-derived hydroxyhydroperoxides on the expression of oxidative stress response genes in human bronchial epithelial cells, Environmental Science: Processes & Impacts, 20, 332-339.
[https://doi.org/10.1039/C7EM00439G]
-
Armstrong, N.C., Chen, Y., Cui, T., Zhang, Y., Christensen, C., Zhang, Z., Turpin, B.J., Chan, M.N., Gold, A., Ault, A.P., Surratt, J.D. (2022) Isoprene epoxydiol-derived sulfated and nonsulfated oligomers suppress particulate mass loss during oxidative aging of secondary organic aerosol, Environmental Science & Technology, 56, 16611-16620.
[https://doi.org/10.1021/acs.est.2c03200]
-
Asa-Awuku, A., Engelhart, G.J., Lee, B.H., Pandis, S.N., Nenes, A. (2009) Relating CCN activity, volatility, and droplet growth kinetics of β-caryophyllene secondary organic aerosol, Atmospheric Chemistry and Physics, 9, 795-812.
[https://doi.org/10.5194/acp-9-795-2009]
-
Asa-Awuku, A., Nenes, A., Gao, S., Flagan, R.C., Seinfeld, J.H. (2010) Water-soluble SOA from alkene ozonolysis: composition and droplet activation kinetics inferences from analysis of CCN activity, Atmospheric Chemistry and Physics, 10, 1585-1597.
[https://doi.org/10.5194/acp-10-1585-2010]
-
Azzi, M., White, S.J., Angove, D.E., Jamie, I.M., Kaduewela, A. (2010) Evaluation of the SAPRC-07 mechanism against CSIRO smog chamber data, Atmospheric Environment, 44, 1707-1713.
[https://doi.org/10.1016/j.atmosenv.2010.02.013]
-
Babar, Z.B., Park, J.-H., Kang, J., Lim, H.-J. (2016) Characterization of a smog chamber for studying formation and physicochemical properties of secondary organic aerosol, Aerosol and Air Quality Research, 16, 3102-3113.
[https://doi.org/10.4209/aaqr.2015.10.0580]
- Bae, G.N., Kim, M.C., Lee, S.B., Song, K.B., Jin, H.C., Moon, K.C. (2003) Design and performance evaluation of the KIST indoor smog chamber, Journal of Korean Society for Atmospheric Environment, 19, 437-449, (in Korean with English abstract).
-
Bandowe, B.A.M., Meusel, H. (2017) Nitrated polycyclic aromatic hydrocarbons (nitro-PAHs) in the environment - a review, Science of The Total Environment, 581, 237-257.
[https://doi.org/10.1016/j.scitotenv.2016.12.115]
-
Barnes, I., Bastian, V., Becker, K.H., Fink, E.H., Zabel, F. (1982) Reactivity studies of organic substances towards hydroxyl radicals under atmospheric conditions, Atmospheric Environment, 16, 545-550.
[https://doi.org/10.1016/0004-6981(82)90163-9]
-
Barnes, I., Becker, K.H., Fink, E.H., Reimer, A., Zabel, F., Niki, H. (1983) Rate constant and products of the reaction CS2 + OH in the presence of O2, International Journal of Chemical Kinetics, 15, 631-645.
[https://doi.org/10.1002/kin.550150705]
-
Bates, J.T., Fang, T., Verma, V., Zeng, L., Weber, R.J., Tolbert, P.E., Abrams, J.Y., Sarnat, S.E., Klein, M., Mulholland, J.A., Russell, A.G. (2019) Review of acellular assays of ambient particulate matter oxidative potential: methods and relationships with composition, sources, and health effects, Environmental Science & Technology, 53, 4003-4019.
[https://doi.org/10.1021/acs.est.8b03430]
-
Beardsley, R., Jang, M., Ori, B., Im, Y., Delcomyn, C.A., Witherspoon, N. (2013) Role of sea salt aerosols in the formation of aromatic secondary organic aerosol: yields and hygroscopic properties, Environmental Chemistry, 10, 167.
[https://doi.org/10.1071/EN13016]
-
Becker, K.H., Wirtz, K. (1989) Gas phase reactions of alkyl nitrates with hydroxyl radicals under tropospheric conditions in comparison with photolysis, Journal of Atmospheric Chemistry, 9, 419-433.
[https://doi.org/10.1007/BF00114754]
-
Beer, T., Carras, J., Worth, D., Coplin, N., Campbell, P., Jalaludin, B., Angove, D., Azzi, M., Brown, S., Campbell, I., Cope, M., Farrell, O., Galbally, I., Haiser, S., Halliburton, B., Hynes, R., Jacyna, D., Keywood, M., Lavrencic, S., Lawson, S., Lee, S., Liepa, I., McGregor, J., Nancarrow, P., Patterson, M., Powell, J., Tibbett, A., Ward, J., White, S., Williams, D., Wood, R. (2011) The health impacts of ethanol blend petrol, Energies, 4, 352-367.
[https://doi.org/10.3390/en4020352]
-
Bianchi, F., Kurtén, T., Riva, M., Mohr, C., Rissanen, M.P., Roldin, P., Berndt, T., Crounse, J.D., Wennberg, P.O., Mentel, T.F., Wildt, J., Junninen, H., Jokinen, T., Kulmala, M., Worsnop, D.R., Thornton, J.A., Donahue, N., Kjaergaard, H.G., Ehn, M. (2019) Highly oxygenated organic molecules (HOM) from gas-phase autoxidation involving peroxy radicals: a key contributor to atmospheric aerosol, Chemical Reviews, 119, 3472-3509.
[https://doi.org/10.1021/acs.chemrev.8b00395]
-
Bloss, C., Wagner, V., Bonzanini, A., Jenkin, M.E., Wirtz, K., Martin-Reviejo, M., Pilling, M.J. (2005a) Evaluation of detailed aromatic mechanisms (MCMv3 and MCMv3.1) against environmental chamber data, Atmospheric Chemistry and Physics, 5, 623-639.
[https://doi.org/10.5194/acp-5-623-2005]
-
Bloss, C., Wagner, V., Jenkin, M.E., Volkamer, R., Bloss, W.J., Lee, J.D., Heard, D.E., Wirtz, K., Martin-Reviejo, M., Rea, G., Wenger, J.C., Pilling, M.J. (2005b) Development of a detailed chemical mechanism (MCMv3.1) for the atmospheric oxidation of aromatic hydrocarbons, Atmospheric Chemistry and Physics, 5, 641-664.
[https://doi.org/10.5194/acp-5-641-2005]
-
Bloss, W.J., Lee, J.D., Bloss, C., Heard, D.E., Pilling, M.J., Wirtz, K., Martin-Reviejo, M., Siese, M. (2004) Validation of the calibration of a laser-induced fluorescence instrument for the measurement of OH radicals in the atmosphere, Atmospheric Chemistry and Physics, 4, 571-583.
[https://doi.org/10.5194/acp-4-571-2004]
-
Borrás, E., Ródenas, M., Vera, T., Gómez, T., Muñoz, A. (2017) Atmospheric degradation of the organothiophosphate insecticide - pirimiphos-methyl, Science of The Total Environment, 579, 1-9.
[https://doi.org/10.1016/j.scitotenv.2016.11.009]
-
Bouzidi, H., Fayad, L., Coeur, C., Houzel, N., Petitprez, D., Faccinetto, A., Wu, J., Tomas, A., Ondráček, J., Schwarz, J., Ždímal, V., Zuend, A. (2022) Hygroscopic growth and CCN activity of secondary organic aerosol produced from dark ozonolysis of γ-terpinene, Science of The Total Environment, 817, 153010.
[https://doi.org/10.1016/j.scitotenv.2022.153010]
- Bunz, H., Möhler, O., Naumann, K.H., Saathoff, H., Schöck, W., Schurath, U. (1996) The novel aerosol chamber facility AIDA: status and first results, Proceeding of the 7th European Symposium on the Physico-Chemical Behaviour of Atmospheric Pollutants, 25.
-
Cao, G., Jang, M. (2010) An SOA model for toluene oxidation in the presence of inorganic aerosols, Environmental Science & Technology, 44, 727-733.
[https://doi.org/10.1021/es901682r]
-
Carter, W., Cockeriii, D., Fitz, D., Malkina, I., Bumiller, K., Sauer, C., Pisano, J., Bufalino, C., Song, C. (2005) A new environmental chamber for evaluation of gas-phase chemical mechanisms and secondary aerosol formation, Atmospheric Environment, 39, 7768-7788.
[https://doi.org/10.1016/j.atmosenv.2005.08.040]
- Carter, W.P., Heo, G., Cocker III, D.R., Nakao, S. (2012) SOA formation: chamber study and model development, Final report to CARB contract 08-326.
-
Carter, W.P.L., Lurmann, F.W. (1991) Evaluation of a detailed gas-phase atmospheric reaction mechanism using environmental chamber data, Atmospheric Environment. Part A. General Topics, 25, 2771-2806.
[https://doi.org/10.1016/0960-1686(91)90206-M]
-
Chandramouli, B., Kamens, R.M. (2001) The photochemical formation and gas-particle partitioning of oxidation products of decamethyl cyclopentasiloxane and decamethyl tetrasiloxane in the atmosphere, Atmospheric Environment, 35, 87-95.
[https://doi.org/10.1016/S1352-2310(00)00289-2]
-
Charan, S.M., Kong, W., Flagan, R.C., Seinfeld, J.H. (2018) Effect of particle charge on aerosol dynamics in teflon environmental chambers, Aerosol Science and Technology, 52, 854-871.
[https://doi.org/10.1080/02786826.2018.1474167]
-
Chen, L., Bao, K., Li, K., Lv, B., Bao, Z., Lin, C., Wu, X., Zheng, C., Gao, X., Cen, K. (2017) Ozone and secondary organic aerosol formation of toluene/NOx irradiations under complex pollution scenarios, Aerosol and Air Quality Research, 17, 1760-1771.
[https://doi.org/10.4209/aaqr.2017.05.0179]
-
Chen, L., Bao, Z., Wu, X., Li, K., Han, L., Zhao, X., Zhang, X., Wang, Z., Azzi, M., Cen, K. (2020) The effects of humidity and ammonia on the chemical composition of secondary aerosols from toluene/NOx photo-oxidation, Science of The Total Environment, 728, 138671.
[https://doi.org/10.1016/j.scitotenv.2020.138671]
-
Chen, T., Chu, B., Ma, Q., Zhang, P., Liu, J., He, H. (2021) Effect of relative humidity on SOA formation from aromatic hydrocarbons: implications from the evolution of gas- and particle-phase species, Science of The Total Environment, 773, 145015.
[https://doi.org/10.1016/j.scitotenv.2021.145015]
-
Chen, T., Liu, Y., Chu, B., Liu, C., Liu, J., Ge, Y., Ma, Q., Ma, J., He, H. (2019a) Differences of the oxidation process and secondary organic aerosol formation at low and high precursor concentrations, Journal of Environmental Sciences, 79, 256-263.
[https://doi.org/10.1016/j.jes.2018.11.011]
-
Chen, T., Liu, Y., Ma, Q., Chu, B., Zhang, P., Liu, C., Liu, J., He, H. (2019b) Significant source of secondary aerosol: formation from gasoline evaporative emissions in the presence of SO2 and NH3, Atmospheric Chemistry and Physics, 19, 8063-8081.
[https://doi.org/10.5194/acp-19-8063-2019]
-
Chen, Y., Sexton, K.G., Jerry, R.E., Surratt, J.D., Vizuete, W. (2015) Assessment of SAPRC07 with updated isoprene chemistry against outdoor chamber experiments, Atmospheric Environment, 105, 109-120.
[https://doi.org/10.1016/j.atmosenv.2015.01.042]
-
Chhabra, P.S., Flagan, R.C., Seinfeld, J.H. (2010) Elemental analysis of chamber organic aerosol using an aerodyne high-resolution aerosol mass spectrometer, Atmospheric Chemistry and Physics, 10, 4111-4131.
[https://doi.org/10.5194/acp-10-4111-2010]
-
Choi, J.E., Bae, G.N., Ju, O.J., Lee, S.B., Moon, K.C., Yoon, S.C. (2006) Effect of light intensity on the photooxidation of toluene-NOx-air mixture, Particle and Aerosol Research, 2, 35-43, (in Korean with English abstract).
[https://doi.org/10.5572/KOSAE.2007.23.3.353]
-
Chowdhury, P.H., He, Q., Lasitza Male, T., Brune, W.H., Rudich, Y., Pardo, M. (2018a) Exposure of lung epithelial cells to photochemically aged secondary organic aerosol shows increased toxic effects, Environmental Science & Technology Letters, 5, 424-430.
[https://doi.org/10.1021/acs.estlett.8b00256]
-
Chu, B., Chen, T., Liu, Y., Ma, Q., Mu, Y., Wang, Y., Ma, J., Zhang, P., Liu, J., Liu, C., Gui, H., Hu, R., Hu, B., Wang, X., Wang, Y., Liu, J., Xie, P., Chen, J., Liu, Q., Jiang, J., Li, J., He, K., Liu, W., Jiang, G., Hao, J., He, H. (2022) Application of smog chambers in atmospheric process studies, National Science Review, 9, nwab103.
[https://doi.org/10.1093/nsr/nwab103]
-
Chu, B., Hao, J., Li, J., Takekawa, H., Wang, K., Jiang, J. (2013) Effects of two transition metal sulfate salts on secondary organic aerosol formation in toluene/NOx photooxidation, Frontiers of Environmental Science & Engineering, 7, 1-9.
[https://doi.org/10.1007/s11783-012-0476-x]
-
Chu, B., Liu, Y., Li, J., Takekawa, H., Liggio, J., Li, S.-M., Jiang, J., Hao, J., He, H. (2014) Decreasing effect and mechanism of FeSO4 seed particles on secondary organic aerosol in α-pinene photooxidation, Environmental Pollution, 193, 88-93.
[https://doi.org/10.1016/j.envpol.2014.06.018]
-
Clergé, A., Le Goff, J., Lopez, C., Ledauphin, J., Delépée, R. (2019) Oxy-PAHs: occurrence in the environment and potential genotoxic/mutagenic risk assessment for human health, Critical Reviews in Toxicology, 49, 302-328.
[https://doi.org/10.1080/10408444.2019.1605333]
-
Cocker, D.R., Flagan, R.C., Seinfeld, J.H. (2001) State-of-the-art chamber facility for studying atmospheric aerosol chemistry, Environmental Science & Technology, 35, 2594-2601.
[https://doi.org/10.1021/es0019169]
-
Crobeddu, B., Aragao-Santiago, L., Bui, L.-C., Boland, S., Baeza Squiban, A. (2017) Oxidative potential of particulate matter 2.5 as predictive indicator of cellular stress, Environmental Pollution, 230, 125-133.
[https://doi.org/10.1016/j.envpol.2017.06.051]
-
Dal Maso, M., Liao, L., Wildt, J., Kiendler-Scharr, A., Kleist, E., Tillmann, R., Sipilä, M., Hakala, J., Lehtipalo, K., Ehn, M., Kerminen, V.-M., Kulmala, M., Worsnop, D., Mentel, T. (2016) A chamber study of the influence of boreal BVOC emissions and sulfuric acid on nanoparticle formation rates at ambient concentrations, Atmospheric Chemistry and Physics, 16, 1955-1970.
[https://doi.org/10.5194/acp-16-1955-2016]
-
Decesari, S., Mircea, M., Cavalli, F., Fuzzi, S., Moretti, F., Tagliavini, E., Facchini, M.C. (2007) Source attribution of water-soluble organic aerosol by nuclear magnetic resonance spectroscopy, Environmental Science & Technology, 41, 2479-2484.
[https://doi.org/10.1021/es061711l]
-
DeMott, P.J., Prenni, A.J., McMeeking, G.R., Sullivan, R.C., Petters, M.D., Tobo, Y., Niemand, M., Möhler, O., Snider, J.R., Wang, Z., Kreidenweis, S.M. (2015) Integrating laboratory and field data to quantify the immersion freezing ice nucleation activity of mineral dust particles, Atmospheric Chemistry and Physics, 15, 393-409.
[https://doi.org/10.5194/acp-15-393-2015]
-
Deng, W., Liu, T., Zhang, Y., Situ, S., Hu, Q., He, Q., Zhang, Z., Lü, S., Bi, X., Wang, X., Boreave, A., George, C., Ding, X., Wang, X. (2017) Secondary organic aerosol formation from photo-oxidation of toluene with NOx and SO2: chamber simulation with purified air versus urban ambient air as matrix, Atmospheric Environment, 150, 67-76.
[https://doi.org/10.1016/j.atmosenv.2016.11.047]
-
Docherty, K.S., Corse, E.W., Jaoui, M., Offenberg, J.H., Kleindienst, T.E., Krug, J.D., Riedel, T.P., Lewandowski, M. (2018) Trends in the oxidation and relative volatility of chamber-generated secondary organic aerosol, Aerosol Science and Technology, 52, 992-1004.
[https://doi.org/10.1080/02786826.2018.1500014]
-
Dodge, M. (2000) Chemical oxidant mechanisms for air quality modeling: critical review, Atmospheric Environment, 34, 2103-2130.
[https://doi.org/10.1016/S1352-2310(99)00461-6]
-
Dovrou, E., Lelieveld, S., Mishra, A., Pöschl, U., Berkemeier, T. (2023) Influence of ambient and endogenous H2O2 on reactive oxygen species concentrations and OH radical production in the respiratory tract, Environmental Science: Atmospheres, 3, 1066-1074.
[https://doi.org/10.1039/D2EA00179A]
-
Doyle, G.J. (1970) Design of a facility (smog chamber) for studying photochemical reactions under simulated tropospheric conditions, Environmental Science & Technology, 4, 907-916.
[https://doi.org/10.1021/es60046a001]
-
Du, L., Xu, Y., Ge, M., Jia, L. (2007) Rate constant for the reaction of ozone with diethyl sulfide, Atmospheric Environment, 41, 7434-7439.
[https://doi.org/10.1016/j.atmosenv.2007.05.041]
-
Duplissy, J., DeCarlo, P.F., Dommen, J., Alfarra, M.R., Metzger, A., Barmpadimos, I., Prevot, A.S.H., Weingartner, E., Tritscher, T., Gysel, M., Aiken, A.C., Jimenez, J.L., Canagaratna, M.R., Worsnop, D.R., Collins, D.R., Tomlinson, J., Baltensperger, U. (2011) Relating hygroscopicity and composition of organic aerosol particulate matter, Atmospheric Chemistry and Physics, 11, 1155-1165.
[https://doi.org/10.5194/acp-11-1155-2011]
-
Duplissy, J., Gysel, M., Alfarra, M.R., Dommen, J., Metzger, A., Prevot, A.S.H., Weingartner, E., Laaksonen, A., Raatikainen, T., Good, N., Turner, S.F., McFiggans, G., Baltensperger, U. (2008) Cloud forming potential of secondary organic aerosol under near atmospheric conditions, Geophysical Research Letters, 35, L03818.
[https://doi.org/10.1029/2007GL031075]
-
Ebersviller, S., Lichtveld, K., Sexton, K.G., Zavala, J., Lin, Y.-H., Jaspers, I., Jeffries, H.E. (2012) Gaseous VOCs rapidly modify particulate matter and its biological effects - Part 2: Complex urban VOCs and model PM, Atmospheric Chemistry and Physics, 12, 12293-12312.
[https://doi.org/10.5194/acp-12-12293-2012]
-
Eddingsaas, N.C., Loza, C.L., Yee, L.D., Chan, M., Schilling, K.A., Chhabra, P.S., Seinfeld, J.H., Wennberg, P.O. (2012a) α-pinene photooxidation under controlled chemical conditions - Part 2: SOA yield and composition in low- and high-NOx environments, Atmospheric Chemistry and Physics, 12, 7413-7427.
[https://doi.org/10.5194/acp-12-7413-2012]
-
Eddingsaas, N.C., Loza, C.L., Yee, L.D., Seinfeld, J.H., Wennberg, P.O. (2012b) α-pinene photooxidation under controlled chemical conditions - Part 1: Gas-phase composition in low- and high-NOx environments, Atmospheric Chemistry and Physics, 12, 6489-6504.
[https://doi.org/10.5194/acp-12-6489-2012]
-
Ehn, M., Kleist, E., Junninen, H., Petäjä, T., Lönn, G., Schobesberger, S., Dal Maso, M., Trimborn, A., Kulmala, M., Worsnop, D.R., Wahner, A., Wildt, J., Mentel, Th.F. (2012) Gas phase formation of extremely oxidized pinene reaction products in chamber and ambient air, Atmospheric Chemistry and Physics, 12, 5113-5127.
[https://doi.org/10.5194/acp-12-5113-2012]
-
Ehn, M., Thornton, J.A., Kleist, E., Sipilä, M., Junninen, H., Pullinen, I., Springer, M., Rubach, F., Tillmann, R., Lee, B. (2014) A large source of low-volatility secondary organic aerosol, Nature, 506, 476-479.
[https://doi.org/10.1038/nature13032]
-
Engelhart, G.J., Asa-Awuku, A., Nenes, A., Pandis, S.N. (2008) CCN activity and droplet growth kinetics of fresh and aged monoterpene secondary organic aerosol, Atmospheric Chemistry and Physics, 8, 3937-3949.
[https://doi.org/10.5194/acp-8-3937-2008]
-
Engelhart, G.J., Moore, R.H., Nenes, A., Pandis, S.N. (2011) Cloud condensation nuclei activity of isoprene secondary organic aerosol, Atmospheric Chemistry and Physics, 116, D02207.
[https://doi.org/10.1029/2010JD014706]
-
Fang, T., Lakey, P.S.J., Weber, R.J., Shiraiwa, M. (2019) Oxidative potential of particulate matter and generation of reactive oxygen species in epithelial lining fluid, Environmental Science & Technology, 53, 12784-12792.
[https://doi.org/10.1021/acs.est.9b03823]
-
Fang, T., Verma, V., Bates, J.T., Abrams, J., Klein, M., Strickland, M.J., Sarnat, S.E., Chang, H.H., Mulholland, J.A., Tolbert, P.E., Russell, A.G., Weber, R.J. (2016) Oxidative potential of ambient water-soluble PM2.5 in the southeastern United States: contrasts in sources and health associations between ascorbic acid (AA) and dithiothreitol (DTT) assays, Atmospheric Chemistry and Physics, 16, 3865-3879.
[https://doi.org/10.5194/acp-16-3865-2016]
-
Fang, T., Zeng, L., Gao, D., Verma, V., Stefaniak, A.B., Weber, R.J. (2017) Ambient size distributions and lung deposition of aerosol dithiothreitol-measured oxidative potential: contrast between soluble and insoluble particles, Environmental Science & Technology, 51, 6802-6811.
[https://doi.org/10.1021/acs.est.7b01536]
-
Farrugia, L.N., Bejan, I., Smith, S.C., Medeiros, D.J., Seakins, P.W. (2015) Revised structure activity parameters derived from new rate coefficient determinations for the reactions of chlorine atoms with a series of seven ketones at 290 K and 1 atm, Chemical Physics Letters, 640, 87-93.
[https://doi.org/10.1016/j.cplett.2015.09.055]
-
Faust, J.A., Wong, J.P.S., Lee, A.K.Y., Abbatt, J.P.D. (2017) Role of aerosol liquid water in secondary organic aerosol formation from volatile organic compounds, Environmental Science & Technology, 51, 1405-1413.
[https://doi.org/10.1021/acs.est.6b04700]
-
Fofie, E.A., Donahue, N.M., Asa-Awuku, A. (2018) Cloud condensation nuclei activity and droplet formation of primary and secondary organic aerosol mixtures, Aerosol Science and Technology, 52, 242-251.
[https://doi.org/10.1080/02786826.2017.1392480]
-
Frey, W., Hu, D., Dorsey, J., Alfarra, M.R., Pajunoja, A., Virtanen, A., Connolly, P., McFiggans, G. (2018) The efficiency of secondary organic aerosol particles acting as ice-nucleating particles under mixed-phase cloud conditions, Atmospheric Chemistry and Physics, 18, 9393-9409.
[https://doi.org/10.5194/acp-18-9393-2018]
-
Frosch, M., Bilde, M., DeCarlo, P.F., Jurányi, Z., Tritscher, T., Dommen, J., Donahue, N.M., Gysel, M., Weingartner, E., Baltensperger, U. (2011) Relating cloud condensation nuclei activity and oxidation level of α-pinene secondary organic aerosols, Journal of Geophysical Research: Atmospheres, 116, D22212.
[https://doi.org/10.1029/2011JD016401]
-
Fuchs, H., Brauers, T., Dorn, H.-P., Harder, H., Häseler, R., Hofzumahaus, A., Holland, F., Kanaya, Y., Kajii, Y., Kubistin, D., Lou, S., Martinez, M., Miyamoto, K., Nishida, S., Rudolf, M., Schlosser, E., Wahner, A., Yoshino, A., Schurath, U. (2010) Formal blind intercomparison of HO2 measurements in the atmosphere simulation chamber SAPHIR during the HOxComp campaign, Atmospheric Chemistry and Physics, 10, 12233-12250.
[https://doi.org/10.5194/acp-10-12233-2010]
-
Fuchs, H., Dorn, H.-P., Bachner, M., Bohn, B., Brauers, T., Gomm, S., Hofzumahaus, A., Holland, F., Nehr, S., Rohrer, F., Tillmann, R., Wahner, A. (2012) Comparison of OH concentration measurements by DOAS and LIF during SAPHIR chamber experiments at high OH reactivity and low NO concentration, Atmospheric Measurement Techniques, 5, 1611-1626.
[https://doi.org/10.5194/amt-5-1611-2012]
-
Fuller, R., Landrigan, P.J., Balakrishnan, K., Bathan, G., Bose-O’Reilly, S., Brauer, M., Caravanos, J., Chiles, T., Cohen, A., Corra, L., Cropper, M., Ferraro, G., Hanna, J., Hanrahan, D., Hu, H., Hunter, D., Janata, G., Kupka, R., Lanphear, B., Lichtveld, M., Martin, K., Mustapha, A., Sanchez-Triana, E., Sandilya, K., Schaefli, L., Shaw, J., Seddon, J., Suk, W., Téllez-Rojo, M.M., Yan, C. (2022) Pollution and health: a progress update, The Lancet Planetary Health, 6, e535-e547.
[https://doi.org/10.1016/S2542-5196(22)00090-0]
-
Gao, D., Ripley, S., Weichenthal, S., Godri Pollitt, K.J. (2020) Ambient particulate matter oxidative potential: chemical determinants, associated health effects, and strategies for risk management, Free Radical Biology and Medicine, 151, 7-25.
[https://doi.org/10.1016/j.freeradbiomed.2020.04.028]
-
Gao, L., Buchholz, A., Li, Z., Song, J., Vallon, M., Jiang, F., Möhler, O., Leisner, T., Saathoff, H. (2023) Volatility of secondary organic aerosol from β-caryophyllene ozonolysis over a wide tropospheric temperature range, Environmental Science & Technology, 57, 8965-8974.
[https://doi.org/10.1021/acs.est.3c01151]
-
Gao, L., Song, J., Mohr, C., Huang, W., Vallon, M., Jiang, F., Leisner, T., Saathoff, H. (2022) Kinetics, SOA yields, and chemical composition of secondary organic aerosol from β-caryophyllene ozonolysis with and without nitrogen oxides between 213 and 313 K, Atmospheric Chemistry and Physics, 22, 6001-6020.
[https://doi.org/10.5194/acp-22-6001-2022]
-
Glowania, M., Rohrer, F., Dorn, H.-P., Hofzumahaus, A., Holland, F., Kiendler-Scharr, A., Wahner, A., Fuchs, H. (2021) Comparison of formaldehyde measurements by Hantzsch, CRDS and DOAS in the SAPHIR chamber, Atmospheric Measurement Techniques, 14, 4239-4253.
[https://doi.org/10.5194/amt-14-4239-2021]
-
Gu, S., Guenther, A., Faiola, C. (2021) Effects of anthropogenic and biogenic volatile organic compounds on los angeles air quality, Environmental Science & Technology, 55, 12191-12201.
[https://doi.org/10.1021/acs.est.1c01481]
-
Gunthe, S.S., King, S.M., Rose, D., Chen, Q., Roldin, P., Farmer, D.K., Jimenez, J.L., Artaxo, P., Andreae, M.O., Martin, S.T., Pöschl, U. (2009) Cloud condensation nuclei in pristine tropical rainforest air of Amazonia: size-resolved measurements and modeling of atmospheric aerosol composition and CCN activity, Atmospheric Chemistry and Physics, 9, 7551-7575.
[https://doi.org/10.5194/acp-9-7551-2009]
-
Hallquist, M., Wängberg, I., Ljungström, E., Barnes, I., Becker, K.-H. (1999) Aerosol and product yields from NO3 radical-initiated oxidation of selected monoterpenes, Environmental Science & Technology, 33, 553-559.
[https://doi.org/10.1021/es980292s]
-
Han, S., Jang, M. (2020) Simulating the impact of gas-wall partitioning on SOA formation using the explicit gas mechanism integrated with aqueous reactions containing electrolytes, Science of The Total Environment, 748, 141360.
[https://doi.org/10.1016/j.scitotenv.2020.141360]
-
Hao, L., Wang, Z., Huang, M., Fang, L., Zhang, W. (2007) Effects of seed aerosols on the growth of secondary organic aerosols from the photooxidation of toluene, Journal of Environmental Sciences, 19, 704-708.
[https://doi.org/10.1016/S1001-0742(07)60117-X]
-
Hazari, M.S., Stratford, K.M., Krantz, Q.T., King, C., Krug, J., Farraj, A.K., Gilmour, M.I. (2018) Comparative cardiopulmonary effects of particulate matter- and ozone-enhanced smog atmospheres in mice, Environmental Science & Technology, 52, 3071-3080.
[https://doi.org/10.1021/acs.est.7b04880]
-
Heald, C.L., Kroll, J.H., Jimenez, J.L., Docherty, K.S., DeCarlo, P.F., Aiken, A.C., Chen, Q., Martin, S.T., Farmer, D.K., Artaxo, P. (2010) A simplified description of the evolution of organic aerosol composition in the atmosphere, Geophysical Research Letters, 37(8).
[https://doi.org/10.1029/2010GL042737]
-
Healy, R.M., Temime, B., Kuprovskyte, K., Wenger, J.C. (2009) Effect of relative humidity on gas/particle partitioning and aerosol mass yield in the photooxidation of p-xylene, Environmental Science & Technology, 43, 1884-1889.
[https://doi.org/10.1021/es802404z]
-
Hess, G.D., Carnovale, F., Cope, M.E., Johnson, G.M. (1992) The evaluation of some photochemical smog reaction mechanisms - I. Temperature and initial composition effects, Atmospheric Environment. Part A. General Topics, 26, 625-641.
[https://doi.org/10.1016/0960-1686(92)90174-J]
-
Hidy, G.M. (2019) Atmospheric Chemistry in a Box or a Bag, Atmosphere, 10, 401.
[https://doi.org/10.3390/atmos10070401]
-
Hiranuma, N., Paukert, M., Steinke, I., Zhang, K., Kulkarni, G., Hoose, C., Schnaiter, M., Saathoff, H., Möhler, O. (2014) A comprehensive parameterization of heterogeneous ice nucleation of dust surrogate: laboratory study with hematite particles and its application to atmospheric models, Atmospheric Chemistry and Physics, 14, 13145-13158.
[https://doi.org/10.5194/acp-14-13145-2014]
-
Hohaus, T., Kuhn, U., Andres, S., Kaminski, M., Rohrer, F., Tillmann, R., Wahner, A., Wegener, R., Yu, Z., Kiendler-Scharr, A. (2016) A new plant chamber facility, PLUS, coupled to the atmosphere simulation chamber SAPHIR, Atmospheric Measurement Techniques, 9, 1247-1259.
[https://doi.org/10.5194/amt-9-1247-2016]
- Hong, Y.D., Han, J.S., Kong, B.J., Lee, S.U., Shin, S.A., Kim, Y.M., Lee, S.J. (2003) A Study on the high-ozone episode and photochemical smog (III), National Institute of Environmental Research (NIER) Report No. 2003-11-683.
-
Huang, M., Zhang, W., Gu, X., Hu, C., Zhao, W., Wang, Z., Fang, L. (2012) Size distribution and chemical composition of secondary organic aerosol formed from Cl-initiated oxidation of toluene, Journal of Environmental Sciences, 24, 860-864.
[https://doi.org/10.1016/S1001-0742(11)60840-1]
-
Huang, W., Saathoff, H., Pajunoja, A., Shen, X., Naumann, K.-H., Wagner, R., Virtanen, A., Leisner, T., Mohr, C. (2018) α-Pinene secondary organic aerosol at low temperature: chemical composition and implications for particle viscosity, Atmospheric Chemistry and Physics, 18, 2883-2898.
[https://doi.org/10.5194/acp-18-2883-2018]
-
Huang, Y., Zhao, R., Charan, S.M., Kenseth, C.M., Zhang, X., Seinfeld, J.H. (2018) Unified theory of vapor-wall mass transport in teflon-walled environmental chambers, Environmental Science & Technology, 52, 2134-2142.
[https://doi.org/10.1021/acs.est.7b05575]
-
Huff Hartz, K.E., Rosenørn, T., Ferchak, S.R., Raymond, T.M., Bilde, M., Donahue, N.M., Pandis, S.N. (2005) Cloud condensation nuclei activation of monoterpene and sesquiterpene secondary organic aerosol, Journal of Geophysical Research: Atmospheres, 110, D14208.
[https://doi.org/10.1029/2004JD005754]
-
Hynes, R., Angove, D., Saunders, S., Haverd, V., Azzi, M. (2005) Evaluation of two MCM v3.1 alkene mechanisms using indoor environmental chamber data, Atmospheric Environment, 39, 7251-7262.
[https://doi.org/10.1016/j.atmosenv.2005.09.005]
-
Iinuma, Y., Keywood, M., Gnauk, T., Herrmann, H. (2009) Diaterebic acid acetate and diaterpenylic acid acetate: atmospheric tracers for secondary organic aerosol formation from 1,8-cineole oxidation, Environmental Science & Technology, 43, 280-285.
[https://doi.org/10.1021/es802141v]
-
Jang, M., Berthold, D.E., Yu, Z., Silva-Sanchez, C., Laughinghouse Iv, H.D., Denslow, N.D., Han, S. (2020) Atmospheric progression of microcystin-lr from cyanobacterial aerosols, Environmental Science & Technology Letters, 7, 740-745.
[https://doi.org/10.1021/acs.estlett.0c00464]
-
Jaoui, M., Leungsakul, S., Kamens, R.M. (2003) Gas and particle products distribution from the reaction of β-caryophyllene with ozone, Journal of Atmospheric Chemistry, 45, 261-287.
[https://doi.org/10.1023/A:1024263430285]
-
Jaoui, M., Sexton, K.G., Kamens, R.M. (2004) Reaction of α-cedrene with ozone: mechanism, gas and particulate products distribution, Atmospheric Environment, 38, 2709-2725.
[https://doi.org/10.1016/j.atmosenv.2004.02.007]
- Jeffries, H., Fox, D., Kamens, R. (1975) Outdoor smog chamber studies. Effect of hydrocarbon reduction on nitrogen dioxide (No. PB-245829), North Carolina University, Chapel Hill (USA), Department of Environmental Sciences and Engineering.
-
Jeffries, H., Fox, D., Kamens, R. (1976) Outdoor smog chamber studies: light effects relative to indoor chambers, Environmental Science & Technology, 10(10), 1006-1011.
[https://doi.org/10.1021/es60121a016]
-
Jeffries, H.E., Fox, D.L., Kamens, R. (1976) Photochemical conversion of NO to NO2 by hydrocarbons in an outdoor chamber, Journal of the Air Pollution Control Association, 26, 480-484.
[https://doi.org/10.1080/00022470.1976.10470274]
-
Ji, Y., Zhao, J., Terazono, H., Misawa, K., Levitt, N.P., Li, Y., Lin, Y., Peng, J., Wang, Y., Duan, L., Pan, B., Zhang, F., Feng, X., An, T., Marrero-Ortiz, W., Secrest, J., Zhang, A.L., Shibuya, K., Molina, M.J., Zhang, R. (2017) Reassessing the atmospheric oxidation mechanism of toluene, Proceedings of the National Academy of Sciences of the United States of America, 114, 8169-8174.
[https://doi.org/10.1073/pnas.1705463114]
-
Jia, L., Xu, Y. (2018) Different roles of water in secondary organic aerosol formation from toluene and isoprene, Atmospheric Chemistry and Physics, 18, 8137-8154.
[https://doi.org/10.5194/acp-18-8137-2018]
-
Jia, L., Xu, Y., Ge, M., Du, L., Zhuang, G. (2009) Smog chamber studies of ozone formation potentials for isopentane, Chinese Science Bulletin, 54, 4624-4632.
[https://doi.org/10.1007/s11434-009-0482-y]
-
Jiang, H., Ahmed, C.S., Canchola, A., Chen, J.Y., Lin, Y.H. (2019) Use of dithiothreitol assay to evaluate the oxidative potential of atmospheric aerosols, Atmosphere, 10(10), 571.
[https://doi.org/10.3390/atmos10100571]
-
Jiang, H., Jang, M., Sabo-Attwood, T., Robinson, S.E. (2016) Oxidative potential of secondary organic aerosols produced from photooxidation of different hydrocarbons using outdoor chamber under ambient sunlight, Atmospheric Environment, 131, 382-389.
[https://doi.org/10.1016/j.atmosenv.2016.02.016]
-
Jiang, H., Jang, M., Yu, Z. (2017) Dithiothreitol activity by particulate oxidizers of SOA produced from photooxidation of hydrocarbons under varied NOx levels, Atmospheric Chemistry and Physics, 17, 9965-9977.
[https://doi.org/10.5194/acp-17-9965-2017]
-
Jiang, J., Carter, W.P.L., Cocker, D.R., Barsanti, K.C. (2020) Development and evaluation of a detailed mechanism for gas-phase atmospheric reactions of furans, ACS Earth and Space Chemistry, 4, 1254-1268.
[https://doi.org/10.1021/acsearthspacechem.0c00058]
-
Ju, O.-J., Bae, G.-N., Choi, J.-E., Lee, S.-B., Ghim, Y.-S., Moon, K.-C., Yoon, S.-C. (2007) Comparison of temperature and light intensity effects on the photooxidation of toluene-NOx-air mixture, Journal of Korean Society for Atmospheric Environment, 23, 353-363, (in Korean with English abstract).
[https://doi.org/10.5572/KOSAE.2007.23.3.353]
-
Jurányi, Z., Gysel, M., Duplissy, J., Weingartner, E., Tritscher, T., Dommen, J., Henning, S., Ziese, M., Kiselev, A., Stratmann, F., George, I., Baltensperger, U. (2009) Influence of gas-to-particle partitioning on the hygroscopic and droplet activation behaviour of α-pinene secondary organic aerosol, Physical Chemistry Chemical Physics, 11, 8091.
[https://doi.org/10.1039/b904162a]
-
Kamens, R.M., Fulcher, J.N., Zhishi, G. (1986) Effects of temperature on wood soot pah decay in atmospheres with sunlight and low NOx, Atmospheric Environment, 20, 1579-1587.
[https://doi.org/10.1016/0004-6981(86)90247-7]
-
Kamens, R.M., Jeffries, H.E., Gery, M.W., Wiener, R.W., Sexton, K.G., Howe, G.B. (1981) The impact of α-pinene on urban smog formation: an outdoor smog chamber study, Atmospheric Environment, 15, 969-981.
[https://doi.org/10.1016/0004-6981(81)90097-4]
-
Kamens, R.M., Rives, G.D., Perry, J.M., Bell, D.A., Paylo, R.F., Goodman, R.G., Claxton, L.D. (1984) Mutagenic changes in dilute wood smoke as it ages and reacts with ozone and nitrogen dioxide. An outdoor chamber study, Environmental Science & Technology, 18, 523-530.
[https://doi.org/10.1021/es00125a008]
-
Kamens, R.M., Zhang, H., Chen, E.H., Zhou, Y., Parikh, H.M., Wilson, R.L., Galloway, K.E., Rosen, E.P. (2011) Secondary organic aerosol formation from toluene in an atmospheric hydrocarbon mixture: water and particle seed effects, Atmospheric Environment, 45, 2324-2334.
[https://doi.org/10.1016/j.atmosenv.2010.11.007]
-
Kamm, S., Möhler, O., Naumann, K.-H., Saathoff, H., Schurath, U. (1999) The heterogeneous reaction of ozone with soot aerosol, Atmospheric Environment, 33, 4651-4661.
[https://doi.org/10.1016/S1352-2310(99)00235-6]
-
Kang, E., Root, M.J., Toohey, D.W., Brune, W.H. (2007) Introducing the concept of Potential Aerosol Mass (PAM), Atmospheric Chemistry and Physics, 7, 5727-5744.
[https://doi.org/10.5194/acp-7-5727-2007]
-
Kanji, Z.A., Sullivan, R.C., Niemand, M., DeMott, P.J., Prenni, A.J., Chou, C., Saathoff, H., Möhler, O. (2019) Heterogeneous ice nucleation properties of natural desert dust particles coated with a surrogate of secondary organic aerosol, Atmospheric Chemistry and Physics, 19, 5091-5110.
[https://doi.org/10.5194/acp-19-5091-2019]
-
Karl, M., Brauers, Th., Dorn, H.-P., Holland, F., Komenda, M., Poppe, D., Rohrer, F., Rupp, L., Schaub, A., Wahner, A. (2004) Kinetic study of the OH-isoprene and O3-isoprene reaction in the atmosphere simulation chamber, SAPHIR, Geophysical Research Letters, 31, L05117.
[https://doi.org/10.1029/2003GL019189]
-
Keywood, M.D., Varutbangkul, V., Bahreini, R., Flagan, R.C., Seinfeld, J.H. (2004) Secondary organic aerosol formation from the ozonolysis of cycloalkenes and related compounds, Environmental Science & Technology, 38, 4157-4164.
[https://doi.org/10.1021/es035363o]
-
Kim, H.C., Kim, S., Kim, B.-U., Jin, C.-S., Hong, S., Park, R., Son, S.-W., Bae, C., Bae, M., Song, C.-K., Stein, A. (2017) Recent increase of surface particulate matter concentrations in the Seoul Metropolitan Area, Korea, Scientific Reports, 7, 4710.
[https://doi.org/10.1038/s41598-017-05092-8]
- Kim, J. (2002) Photochemical reactions of real gas in an indoor smog chamber. MD Thesis, Department of Environmental Engineering, The University of Seoul, (in Korean with English abstract).
-
Kim, J.-H., Oh, I.-H., Park, J.-H., Cheong, H.-K. (2018) Premature deaths attributable to long-term exposure to ambient fine particulate matter in the Republic of Korea, Journal of Korean Medical Science, 33, e251, (in Korean with English abstract).
[https://doi.org/10.3346/jkms.2018.33.e251]
-
Kim, M., Park, T., Lee, J.W., Lee, S.H., Song, M., Park, J., Bae, M.-S., Lee, T., Park, H.M. (2021) Assessment of NO reduction using TiO2 in large-scale smog chamber, Journal of Korean Society for Atmospheric Environment, 37, 946-955, (in Korean with English abstract).
[https://doi.org/10.5572/KOSAE.2021.37.6.946]
- Kim, M.C., Bae, G.-N., Moon, K.-C., Park, J.-Y. (2004) Formation and growth of atmospheric aerosols by water vapor reactions in an indoor smog chamber, Journal of Korean Society for Atmospheric Environment, 20, 161-174, (in Korean with English abstract).
-
Kim, M.-G., Lee, S.-J., Park, D., Kim, C., Lee, K.-H., Hwang, J. (2020) Relationship between the actual fine dust concentration and media exposure that influenced the changes in outdoor activity behavior in South Korea, Scientific Reports, 10, 12006.
[https://doi.org/10.1038/s41598-020-68580-4]
-
King, S.M., Rosenoern, T., Shilling, J.E., Chen, Q., Martin, S.T. (2009) Increased cloud activation potential of secondary organic aerosol for atmospheric mass loadings, Atmospheric Chemistry and Physics, 9, 2959-2971.
[https://doi.org/10.5194/acp-9-2959-2009]
-
King, S.M., Rosenoern, T., Shilling, J.E., Chen, Q., Martin, S.T. (2007) Cloud condensation nucleus activity of secondary organic aerosol particles mixed with sulfate, Geophysical Research Letters, 34, L24806.
[https://doi.org/10.1029/2007GL030390]
-
Kleindienst, T.E., Edney, E.O., Namie, G.R., Claxton, L.D. (1986) The mutagenic activity of irradiated mixtures in the presence of diethylhydroxylamine, Atmospheric Environment, 20, 971-978.
[https://doi.org/10.1016/0004-6981(86)90281-7]
-
Klotz, B., Sørensen, S., Barnes, I., Becker, K.H., Etzkorn, T., Volkamer, R., Platt, U., Wirtz, K., Martín-Reviejo, M. (1998) Atmospheric oxidation of toluene in a large-volume outdoor photoreactor: in situ determination of ring-retaining product yields, The Journal of Physical Chemistry A, 102, 10289-10299.
[https://doi.org/10.1021/jp982719n]
-
Kramer, A.J., Rattanavaraha, W., Zhang, Z., Gold, A., Surratt, J.D., Lin, Y.-H. (2016) Assessing the oxidative potential of isoprene-derived epoxides and secondary organic aerosol, Atmospheric Environment, 130, 211-218.
[https://doi.org/10.1016/j.atmosenv.2015.10.018]
-
Krevelen, D.V., Baans, C.M.E. (1950) The elimination of carbon monoxide from synthesis gases by absorption in cuprous salt solutions, The Journal of Physical Chemistry, 54, 370-390.
[https://doi.org/10.1021/j150477a011]
-
Kroll, J.H., Ng, N.L., Murphy, S.M., Flagan, R.C., Seinfeld, J.H. (2006) Secondary organic aerosol formation from isoprene photooxidation, Environmental Science & Technology, 40, 1869-1877.
[https://doi.org/10.1021/es0524301]
-
Kuang, Y., Shang, J. (2020) Changes in light absorption by brown carbon in soot particles due to heterogeneous ozone aging in a smog chamber, Environmental Pollution, 266, 115273.
[https://doi.org/10.1016/j.envpol.2020.115273]
-
Lakey, P.S.J., Berkemeier, T., Tong, H., Arangio, A.M., Lucas, K., Pöschl, U., Shiraiwa, M. (2016) Chemical exposure-response relationship between air pollutants and reactive oxygen species in the human respiratory tract, Scientific Reports, 6, 32916.
[https://doi.org/10.1038/srep32916]
-
Lambe, A.T., Chhabra, P.S., Onasch, T.B., Brune, W.H., Hunter, J.F., Kroll, J.H., Cummings, M.J., Brogan, J.F., Parmar, Y., Worsnop, D.R., Kolb, C.E., Davidovits, P. (2015) Effect of oxidant concentration, exposure time, and seed particles on secondary organic aerosol chemical composition and yield, Atmospheric Chemistry and Physics, 15, 3063-3075.
[https://doi.org/10.5194/acp-15-3063-2015]
-
Lambe, A.T., Onasch, T.B., Massoli, P., Croasdale, D.R., Wright, J.P., Ahern, A.T., Williams, L.R., Worsnop, D.R., Brune, W.H., Davidovits, P. (2011) Laboratory studies of the chemical composition and cloud condensation nuclei (CCN) activity of secondary organic aerosol (SOA) and oxidized primary organic aerosol (OPOA), Atmospheric Chemistry and Physics, 11, 8913-8928.
[https://doi.org/10.5194/acp-11-8913-2011]
-
Lamkaddam, H., Gratien, A., Pangui, E., Cazaunau, M., Picquet-Varrault, B., Doussin, J.-F. (2017) High-NOx photooxidation of n-dodecane: temperature dependence of SOA formation, Environmental Science & Technology, 51, 192-201.
[https://doi.org/10.1021/acs.est.6b03821]
-
Lathem, T.L., Nenes, A. (2011) Water vapor depletion in the DMT continuous-flow CCN chamber: effects on supersaturation and droplet growth, Aerosol Science and Technology, 45, 604-615.
[https://doi.org/10.1080/02786826.2010.551146]
-
Latimer, H.K., Kamens, R.M., Chandra, G. (1998) The atmospheric partitioning of decamethylcyclopentasiloxane (D5) and 1-hydroxynonamethylcyclopentasiloxane (D4TOH) on different types of atmospheric particles, Chemosphere, 36, 2401-2414.
[https://doi.org/10.1016/S0045-6535(97)10209-0]
-
Lavigne, É., Burnett, R.T., Stieb, D.M., Evans, G.J., Godri Pollitt, K.J., Chen, H., Van Rijswijk, D., Weichenthal, S. (2018) Fine particulate air pollution and adverse birth outcomes: effect modification by regional nonvolatile oxidative potential, Environmental Health Perspectives, 126, 077012.
[https://doi.org/10.1289/EHP2535]
-
Lee, A., Goldstein, A.H., Keywood, M.D., Gao, S., Varutbangkul, V., Bahreini, R., Ng, N.L., Flagan, R.C., Seinfeld, J.H. (2006a) Gas-phase products and secondary aerosol yields from the ozonolysis of ten different terpenes, Atmospheric Chemistry and Physics, 111, D07302.
[https://doi.org/10.1029/2005JD006437]
-
Lee, A., Goldstein, A.H., Kroll, J.H., Ng, N.L., Varutbangkul, V., Flagan, R.C., Seinfeld, J.H. (2006b) Gas-phase products and secondary aerosol yields from the photooxidation of 16 different terpenes, Atmospheric Chemistry and Physics, 111, D17305.
[https://doi.org/10.1029/2006JD007050]
-
Lee, S., Jang, M., Kamens, R.M. (2004) SOA formation from the photooxidation of α-pinene in the presence of freshly emitted diesel soot exhaust, Atmospheric Environment, 38, 2597-2605.
[https://doi.org/10.1016/j.atmosenv.2003.12.041]
- Lee, S.-B., Bae, G.-N., Kim, M.C., Lee, Y.-M., Moon, K.-C. (2006a) Effect of relative humidity on the growth of atmospheric aerosols in winter, Particle and Aerosol Research, 2, 69-81, (in Korean with English abstract).
- Lee, S.-B., Bae, G.-N., Moon, K.-C., Choi, M. (2006b) Effect of diesel exhaust on the photochemical reactions of ambient air, Particle and Aerosol Research, 2, 127-140, (in Korean with English abstract).
-
Lee, S.-B., Bae, G.-N., Lee, Y.-M., Moon, K.-C. (2013) Wall contamination of teflon bags used as a photochemical reaction chamber of ambient air, Particle and Aerosol Research, 9, 149-161, (in Korean with English abstract).
[https://doi.org/10.11629/JPAAR.2013.9.3.149]
-
Lee, S.-B., Bae, G.-N., Lee, Y.-M., Moon, K.-C., Choi, M. (2010) Correlation between light intensity and ozone formation for photochemical smog in urban air of Seoul, Aerosol and Air Quality Research, 10, 540-549.
[https://doi.org/10.4209/aaqr.2010.05.0036]
- Lee, S.-B., Bae, G.-N., Moon, K.-C. (2004) Aerosol wall loss in teflon film chambers filled with ambient air, Journal of Korean Society for Atmospheric Environment, 20, 35-41, (in Korean with English abstract).
-
Leone, J.A., Flagan, R.C., Grosjean, D., Seinfeld, J.H. (1985) An outdoor smog chamber and modeling study of toluene-NOx photooxidation, International Journal of Chemical Kinetics, 17, 177-216.
[https://doi.org/10.1002/kin.550170206]
-
Leungsakul, S., Jaoui, M., Kamens, R.M. (2005) Kinetic mechanism for predicting secondary organic aerosol formation from the reaction of d-limonene with ozone, Environmental Science & Technology, 39, 9583-9594.
[https://doi.org/10.1021/es0492687]
-
Li, J., Li, H., Li, K., Chen, Y., Zhang, H., Zhang, X., Wu, Z., Liu, Y., Wang, X., Wang, W., Ge, M. (2021a) Enhanced secondary organic aerosol formation from the photo-oxidation of mixed anthropogenic volatile organic compounds, Atmospheric Chemistry and Physics, 21, 7773-7789.
[https://doi.org/10.5194/acp-21-7773-2021]
-
Li, J., Li, H., Wang, X., Wang, W., Ge, M., Zhang, H., Zhang, X., Li, K., Chen, Y., Wu, Z., Chai, F., Meng, F., Mu, Y., Mellouki, A., Bi, F., Zhang, Y., Wu, L., Liu, Y. (2021b) A large-scale outdoor atmospheric simulation smog chamber for studying atmospheric photochemical processes: characterization and preliminary application, Journal of Environmental Sciences, 102, 185-197.
[https://doi.org/10.1016/j.jes.2020.09.015]
-
Li, J., Li, K., Zhang, H., Zhang, X., Ji, Y., Chu, W., Kong, Y., Chu, Y., Ren, Y., Zhang, Y., Zhang, H., Gao, R., Wu, Z., Bi, F., Chen, X., Wang, X., Wang, W., Li, H., Ge, M. (2022) Effects of OH radical and SO2 concentrations on photochemical reactions of mixed anthropogenic organic gases, Atmospheric Chemistry and Physics, 22, 10489-10504.
[https://doi.org/10.5194/acp-22-10489-2022]
-
Li, K., Chen, L., Han, K., Lv, B., Bao, K., Wu, X., Gao, X., Cen, K. (2017) Smog chamber study on aging of combustion soot in isoprene/SO2/NOx system: changes of mass, size, effective density, morphology and mixing state, Atmospheric Research, 184, 139-148.
[https://doi.org/10.1016/j.atmosres.2016.10.011]
-
Li, K., Lin, C., Geng, C., White, S., Chen, L., Bao, Z., Zhang, X., Zhao, Y., Han, L., Yang, W., Azzi, M., (2020a) Characterization of a new smog chamber for evaluating SAPRC gas-phase chemical mechanism, Journal of Environmental Sciences, 95, 14-22.
[https://doi.org/10.1016/j.jes.2020.03.028]
-
Li, K., White, S., Zhao, B., Geng, C., Halliburton, B., Wang, Z., Zhao, Y., Yu, H., Yang, W., Bai, Z., Azzi, M. (2020b) Evaluation of a new chemical mechanism for 2-amino-2-methyl-1-propanol in a reactive environment from CSIRO smog chamber experiments, Environmental Science & Technology, 54, 9844-9853.
[https://doi.org/10.1021/acs.est.9b07669]
-
Li, K., Zhang, X., Zhao, B., Bloss, W.J., Lin, C., White, S., Yu, H., Chen, L., Geng, C., Yang, W., Azzi, M., George, C., Bai, Z. (2022) Suppression of anthropogenic secondary organic aerosol formation by isoprene, npj Climate and Atmospheric Science, 5, 12.
[https://doi.org/10.1038/s41612-022-00233-x]
-
Li, Q., Jiang, J., Afreh, I.K., Barsanti, K.C., Cocker III, D.R. (2022) Secondary organic aerosol formation from camphene oxidation: measurements and modeling, Atmospheric Chemistry and Physics, 22, 3131-3147.
[https://doi.org/10.5194/acp-22-3131-2022]
-
Li, W., Cocker, D.R. (2018a) Secondary organic aerosol and ozone formation from photo-oxidation of unburned diesel fuel in a surrogate atmospheric environment, Atmospheric Environment, 184, 17-23.
[https://doi.org/10.1016/j.atmosenv.2018.03.059]
-
Li, W., Li, L., Chen, C., Kacarab, M., Peng, W., Price, D., Xu, J., Cocker, D.R. (2018b) Potential of select intermediate-volatility organic compounds and consumer products for secondary organic aerosol and ozone formation under relevant urban conditions, Atmospheric Environment, 178, 109-117.
[https://doi.org/10.1016/j.atmosenv.2017.12.019]
-
Liang, P., Mu, Y.J., Daële, V., Mellouki, A. (2011) Kinetic studies of Cl reactions with 3-buten-1-ol and 2-buten-1-ol over the temperature range 298-363K, Chemical Physics Letters, 502, 154-158.
[https://doi.org/10.1016/j.cplett.2010.12.058]
-
Lim, Y.B., Lee, S.-B., Kim, H., Kim, J.Y., Bae, G.-N. (2016) Review of recent smog chamber studies for secondary organic aerosol, Journal of Korean Society for Atmospheric Environment, 32, 131-157, (in Korean with English abstract).
[https://doi.org/10.5572/KOSAE.2016.32.2.131]
-
Liu, C., Chen, T., Liu, Y., Liu, J., He, H., Zhang, P. (2019) Enhancement of secondary organic aerosol formation and its oxidation state by SO2 during photooxidation of 2-methoxyphenol, Atmospheric Chemistry and Physics, 19, 2687-2700.
[https://doi.org/10.5194/acp-19-2687-2019]
-
Liu, J., Chu, B., Chen, T., Liu, C., Wang, L., Bao, X., He, H. (2018) Secondary organic aerosol formation from ambient air at an urban site in beijing: effects of OH exposure and precursor concentrations, Environmental Science & Technology, 52, 6834-6841.
[https://doi.org/10.1021/acs.est.7b05701]
-
Liu, P., Song, M., Zhao, T., Gunthe, S.S., Ham, S., He, Y., Qin, Y.M., Gong, Z., Amorim, J.C., Bertram, A.K., Martin, S.T. (2018) Resolving the mechanisms of hygroscopic growth and cloud condensation nuclei activity for organic particulate matter, Nature Communications, 9, 4076.
[https://doi.org/10.1038/s41467-018-06622-2]
-
Liu, S., Jiang, X., Tsona, N.T., Lv, C., Du, L. (2019) Effects of NOx, SO2 and RH on the SOA formation from cyclohexene photooxidation, Chemosphere, 216, 794-804.
[https://doi.org/10.1016/j.chemosphere.2018.10.180]
-
Liu, T., Huang, D.D., Li, Z., Liu, Q., Chan, M., Chan, C.K. (2018) Comparison of secondary organic aerosol formation from toluene on initially wet and dry ammonium sulfate particles at moderate relative humidity, Atmospheric Chemistry and Physics, 18, 5677-5689.
[https://doi.org/10.5194/acp-18-5677-2018]
-
Liu, T., Wang, X., Hu, Q., Deng, W., Zhang, Y., Ding, X., Fu, X., Bernard, F., Zhang, Z., Lü, S., He, Q., Bi, X., Chen, J., Sun, Y., Yu, J., Peng, P., Sheng, G., Fu, J. (2016) Formation of secondary aerosols from gasoline vehicle exhaust when mixing with SO2, Atmospheric Chemistry and Physics, 16, 675-689.
[https://doi.org/10.5194/acp-16-675-2016]
-
Loza, C.L., Chan, A.W.H., Galloway, M.M., Keutsch, F.N., Flagan, R.C., Seinfeld, J.H. (2010) Characterization of vapor wall loss in laboratory chambers, Environmental Science & Technology, 44, 5074-5078.
[https://doi.org/10.1021/es100727v]
-
Lu, Z., Hao, J., Hu, L., Takekawa, H. (2008) The compaction of soot particles generated by spark discharge in the propene ozonolysis system, Journal of Aerosol Science, 39, 897-903.
[https://doi.org/10.1016/j.jaerosci.2008.06.001]
-
Lu, Z., Hao, K., Li, J., Takekawa, H. (2009). Effect of highly concentrated dry (NH4)2SO4 seed aerosols on ozone and secondary organic aerosol formation in aromatic hydrocarbon/NOx photooxidation systems, Atmospheric Aerosols, Chapter 8, 111-126.
[https://doi.org/10.1021/bk-2009-1005.ch008]
-
Lugo, P.L., Straccia, V.G., Rivela, C.B., Patroescu-Klotz, I., Illmann, N., Teruel, M.A., Wiesen, P., Blanco, M.B. (2022) Diurnal photodegradation of fluorinated diketones (FDKs) by OH radicals using different atmospheric simulation chambers: role of keto-enol tautomerization on reactivity, Chemosphere, 286, 131562.
[https://doi.org/10.1016/j.chemosphere.2021.131562]
-
Lutes, C.C., Charles, M.J., Odum, J.R., Kamens, R.M. (1992) Chamber aging studies on the atmospheric stability of polybrominated dibenzo-p-dioxins and dibenzofurans, Environmental Science & Technology, 26, 991-998.
[https://doi.org/10.1021/es00029a019]
-
Ma, W., Liu, Y., Zhang, Y., Feng, Z., Zhan, J., Hua, C., Ma, L., Guo, Y., Zhang, Y., Zhou, W., Yan, C., Chu, B., Chen, T., Ma, Q., Liu, C., Kulmala, M., Mu, Y., He, H. (2022) A new type of quartz smog chamber: design and characterization, Environmental Science & Technology, 56, 2181-2190.
[https://doi.org/10.1021/acs.est.1c06341]
-
Mao, J., Ren, X., Brune, W.H., Olson, J.R., Crawford, J.H., Fried, A., Huey, L.G., Cohen, R.C., Heikes, B., Singh, H.B., Blake, D.R., Sachse, G.W., Diskin, G.S., Hall, S.R., Shetter, R.E. (2009) Airborne measurement of OH reactivity during INTEX-B, Atmospheric Chemistry and Physics, 9, 163-173.
[https://doi.org/10.5194/acp-9-163-2009]
-
Massoli, P., Lambe, A.T., Ahern, A.T., Williams, L.R., Ehn, M., Mikkilä, J., Canagaratna, M.R., Brune, W.H., Onasch, T.B., Jayne, J.T., Petäjä, T., Kulmala, M., Laaksonen, A., Kolb, C.E., Davidovits, P., Worsnop, D.R. (2010) Relationship between aerosol oxidation level and hygroscopic properties of laboratory generated secondary organic aerosol (SOA) particles, Geophysical Research Letters, 37, L24801.
[https://doi.org/10.1029/2010GL045258]
-
McGee Hargrove, M., Snow, S.J., Luebke, R.W., Wood, C.E., Krug, J.D., Krantz, Q.T., King, C., Copeland, C.B., McCullough, S.D., Gowdy, K.M., Kodavanti, U.P., Gilmour, M.I., Gavett, S.H. (2018) Effects of simulated smog atmospheres in rodent models of metabolic and immunologic dysfunction, Environmental Science & Technology, 52, 3062-3070.
[https://doi.org/10.1021/acs.est.7b06534]
-
Molteni, U., Bianchi, F., Klein, F., El Haddad, I., Frege, C., Rossi, M.J., Dommen, J., Baltensperger, U. (2018) Formation of highly oxygenated organic molecules from aromatic compounds, Atmospheric Chemistry and Physics, 18, 1909-1921.
[https://doi.org/10.5194/acp-18-1909-2018]
-
Morino, Y., Sadanaga, Y., Sato, K., Sakamoto, Y., Muraoka, T., Miyatake, K., Li, J., Kajii, Y. (2023) Direct evaluation of the ozone production regime in smog chamber experiments, Atmospheric Environment, 309, 119889.
[https://doi.org/10.1016/j.atmosenv.2023.119889]
-
Morriss, F.V., Bolze, C., Goodwin, J.T., King, F. (1957) Smog experiments in large chambers. equipment and procedure, Industrial & Engineering Chemistry, 49, 1249-1250.
[https://doi.org/10.1021/ie50572a028]
-
Mu, Q., Shiraiwa, M., Octaviani, M., Ma, N., Ding, A., Su, H., Lammel, G., Pöschl, U., Cheng, Y. (2018) Temperature effect on phase state and reactivity controls atmospheric multiphase chemistry and transport of PAHs, Science Advances, 4, eaap7314.
[https://doi.org/10.1126/sciadv.aap7314]
-
Muñoz, A., Borrás, E., Ródenas, M., Vera, T., Pedersen, H.A. (2018) Atmospheric oxidation of a thiocarbamate herbicide used in winter cereals, Environmental Science & Technology, 52, 9136-9144.
[https://doi.org/10.1021/acs.est.8b02157]
-
Muñoz, A., Ródenas, M., Borrás, E., Vázquez, M., Vera, T. (2014a) The gas-phase degradation of chlorpyrifos and chlorpyrifos-oxon towards OH radical under atmospheric conditions, Chemosphere, 111, 522-528.
[https://doi.org/10.1016/j.chemosphere.2014.04.087]
-
Muñoz, A., Vera, T., Ródenas, M., Borrás, E., Mellouki, A., Treacy, J., Sidebottom, H. (2014b) Gas-phase degradation of the herbicide ethalfluralin under atmospheric conditions, Chemosphere, 95, 395-401.
[https://doi.org/10.1016/j.chemosphere.2013.09.053]
-
Nelson, P.F., Quigley, S.M. (1983) The m,p-xylenes:ethylbenzene ratio. A technique for estimating hydrocarbon age in ambient atmospheres, Atmospheric Environment, 17, 659-662.
[https://doi.org/10.1016/0004-6981(83)90141-5]
-
Newland, M.J., Jenkin, M.E., Rickard, A.R. (2017) Elucidating the fate of the OH-adduct in toluene oxidation under tropospheric boundary layer conditions, Proceedings of the National Academy of Sciences of the United States of America, 114.
[https://doi.org/10.1073/pnas.1713678114]
-
Ng, N.L., Kroll, J.H., Chan, A.W.H., Chhabra, P.S., Flagan, R.C., Seinfeld, J.H. (2007) Secondary organic aerosol formation from m-xylene, toluene, and benzene, Atmospheric Chemistry and Physics, 7, 3909-3922.
[https://doi.org/10.5194/acp-7-3909-2007]
- Nie, J.S., Qin, M., Yang, Y., Zhang, W.J. (2002) The structure and performance of a kind of photo chemical smog chamber, Chinese Journal of Atomic and Molecular Physics, 19, 186-190, (in Chinese).
-
O’Dowd, C.D., Jimenez, J.L., Bahreini, R., Flagan, R.C., Seinfeld, J.H., Hämeri, K., Pirjola, L., Kulmala, M., Jennings, S.G., Hoffmann, T. (2002) Marine aerosol formation from biogenic iodine emissions, Nature, 417, 632-636.
[https://doi.org/10.1038/nature00775]
-
Organisation for Economic Co-operation and Development (OECD) (2012) OECD Environmental Outlook to 2050: The Consequences of Inaction, OECD Environmental Outlook.
[https://doi.org/10.1787/9789264122246-en]
-
Øvrevik, J. (2019) Oxidative potential versus biological effects: a review on the relevance of cell-free/abiotic assays as predictors of toxicity from airborne particulate matter, International Journal of Molecular Sciences, 20, 4772.
[https://doi.org/10.3390/ijms20194772]
-
Park, J., Jang, M., Yu, Z. (2017) Heterogeneous photo-oxidation of SO2 in the presence of two different mineral dust particles: gobi and arizona dust, Environmental Science & Technology, 51, 9605-9613.
[https://doi.org/10.1021/acs.est.7b00588]
-
Park, J.-H., Babar, Z.B., Baek, S.J., Kim, H.S., Lim, H.-J. (2017) Effects of NOx on the molecular composition of secondary organic aerosol formed by the ozonolysis and photooxidation of α-pinene, Atmospheric Environment, 166, 263-275.
[https://doi.org/10.1016/j.atmosenv.2017.07.022]
-
Park, J.Y., Jang, M. (2016) Heterogeneous photooxidation of sulfur dioxide in the presence of airborne mineral dust particles, RSC Advances, 6, 58617-58627.
[https://doi.org/10.1039/C6RA09601H]
-
Park, M., Joo, H.S., Lee, K., Jang, M., Kim, S.D., Kim, I., Borlaza, L.J.S., Lim, H., Shin, H., Chung, K.H., Choi, Y.-H., Park, S.G., Bae, M.-S., Lee, J., Song, H., Park, K. (2018) Differential toxicities of fine particulate matters from various sources, Scientific Reports, 8, 17007.
[https://doi.org/10.1038/s41598-018-35398-0]
-
Paulot, F., Crounse, J.D., Kjaergaard, H.G., Kroll, J.H., Seinfeld, J.H., Wennberg, P.O. (2009b) Isoprene photooxidation: new insights into the production of acids and organic nitrates, Atmospheric Chemistry and Physics, 9, 1479-1501.
[https://doi.org/10.5194/acp-9-1479-2009]
-
Paulot, F., Crounse, J.D., Kjaergaard, H.G., Kürten, A., St. Clair, J.M., Seinfeld, J.H., Wennberg, P.O. (2009a) Unexpected epoxide formation in the gas-phase photooxidation of isoprene, Science, 325, 730-733.
[https://doi.org/10.1126/science.1172910]
-
Petters, M.D., Kreidenweis, S.M. (2007) A single parameter representation of hygroscopic growth and cloud condensation nucleus activity, Atmospheric Chemistry and Physics, 7, 1961-1971.
[https://doi.org/10.5194/acp-7-1961-2007]
-
Petters, M.D., Wex, H., Carrico, C.M., Hallbauer, E., Massling, A., McMeeking, G.R., Poulain, L., Wu, Z., Kreidenweis, S.M., Stratmann, F. (2009) Towards closing the gap between hygroscopic growth and activation for secondary organic aerosol - Part 2: Theoretical approaches, Atmospheric Chemistry and Physics, 9, 3999-4009.
[https://doi.org/10.5194/acp-9-3999-2009]
-
Picquet-Varrault, B., Doussin, J.-F., Durand-Jolibois, R., Pirali, O., Carlier, P., Fittschen, C. (2002) Kinetic and mechanistic study of the atmospheric oxidation by OH radicals of allyl acetate, Environmental Science & Technology, 36, 4081-4086.
[https://doi.org/10.1021/es0200138]
-
Pietrogrande, M.C., Bacco, D., Trentini, A., Russo, M. (2021) Effect of filter extraction solvents on the measurement of the oxidative potential of airborne PM2.5, Environmental Science and Pollution Research, 28, 29551-29563.
[https://doi.org/10.1007/s11356-021-12604-7]
- Pitts Jr, J.N. (1977) Mechanisms of photochemical reactions in urban air. Volume I. Chemistry studies. Final report 1 Dec 71-1 Dec 74. California University, Riverside (USA). Statewide Air Pollution Research Center.
- Pitts Jr, J.N., Darnall, K.R., Winer, A.M., McAfee, J.M. (1977) Mechanisms of Photochemical Reactions in Urban Air-Volume II. Chamber Studies. US Environmental Protection Agency Report (US EPA) No. EPA-600/3-77-014b.
-
Prenni, A.J., Petters, M.D., Kreidenweis, S.M., DeMott, P.J., Ziemann, P.J. (2007) Cloud droplet activation of secondary organic aerosol, Journal of Geophysical Research: Atmospheres, 112, D10223.
[https://doi.org/10.1029/2006JD007963]
-
Pye, H.O.T., Murphy, B.N., Xu, L., Ng, N.L., Carlton, A.G., Guo, H., Weber, R., Vasilakos, P., Appel, K.W., Budisulistiorini, S.H., Surratt, J.D., Nenes, A., Hu, W., Jimenez, J.L., Isaacman-VanWertz, G., Misztal, P.K., Goldstein, A.H. (2017) On the implications of aerosol liquid water and phase separation for organic aerosol mass, Atmospheric Chemistry and Physics, 17, 343-369.
[https://doi.org/10.5194/acp-17-343-2017]
-
Raff, J.D., Finlayson-Pitts, B.J. (2010) Hydroxyl radical quantum yields from isopropyl nitrite photolysis in air, Environmental Science & Technology, 44(21), 8150-8155.
[https://doi.org/10.1021/es102218d]
-
Rattanavaraha, W., Rosen, E., Zhang, H., Li, Q., Pantong, K., Kamens, R.M. (2011) The reactive oxidant potential of different types of aged atmospheric particles: an outdoor chamber study, Atmospheric Environment, 45, 3848-3855.
[https://doi.org/10.1016/j.atmosenv.2011.04.002]
-
Ren, Y., Bernard, F., Daële, V., Mellouki, A. (2019) Atmospheric fate and impact of perfluorinated butanone and pentanone, Environmental Science & Technology, 53, 8862-8871.
[https://doi.org/10.1021/acs.est.9b02974]
-
Riva, M., Budisulistiorini, S.H., Zhang, Z., Gold, A., Surratt, J.D. (2016) Chemical characterization of secondary organic aerosol constituents from isoprene ozonolysis in the presence of acidic aerosol, Atmospheric Environment, 130, 5-13.
[https://doi.org/10.1016/j.atmosenv.2015.06.027]
-
Riva, M., Healy, R.M., Flaud, P.-M., Perraudin, E., Wenger, J.C., Villenave, E. (2015) Gas- and particle-phase products from the chlorine-initiated oxidation of polycyclic aromatic hydrocarbons, The Journal of Physical Chemistry A, 119, 11170-11181.
[https://doi.org/10.1021/acs.jpca.5b04610]
-
Roberts, G.C., Nenes, A. (2005) A continuous-flow streamwise thermal-gradient CCN chamber for atmospheric measurements, Aerosol Science and Technology, 39, 206-221.
[https://doi.org/10.1080/027868290913988]
-
Rose, A.H., Brandt, C.S. (1960) Environmental irradiation test facility, Journal of the Air Pollution Control Association, 10, 331-335.
[https://doi.org/10.1080/00022470.1960.10467941]
-
Rose, D., Gunthe, S.S., Mikhailov, E., Frank, G.P., Dusek, U., Andreae, M.O., Pöschl, U. (2008) Calibration and measurement uncertainties of a continuous-flow cloud condensation nuclei counter (DMT-CCNC): CCN activation of ammonium sulfate and sodium chloride aerosol particles in theory and experiment, Atmospheric Chemistry and Physics, 8, 1153-1179.
[https://doi.org/10.5194/acp-8-1153-2008]
-
Rule, A.M., Koehler, K.A. (2022) Particle constituents and oxidative potential: insights into differential fine particulate matter toxicity, American Journal of Respiratory and Critical Care Medicine, 206, 1310-1312.
[https://doi.org/10.1164/rccm.202208-1513ED]
-
Russell, L.M., Bahadur, R., Ziemann, P.J. (2011) Identifying organic aerosol sources by comparing functional group composition in chamber and atmospheric particles, Proceedings of the National Academy of Sciences of the United States of America, 108, 3516-3521.
[https://doi.org/10.1073/pnas.1006461108]
-
Saathoff, H., Naumann, K.-H., Möhler, O., Jonsson, Å.M., Hallquist, M., Kiendler-Scharr, A., Mentel, Th.F., Tillmann, R., Schurath, U. (2009) Temperature dependence of yields of secondary organic aerosols from the ozonolysis of α-pinene and limonene, Atmospheric Chemistry and Physics, 9, 1551-1577.
[https://doi.org/10.5194/acp-9-1551-2009]
-
Sakamoto, Y., Kohno, N., Ramasamy, S., Sato, K., Morino, Y., Kajii, Y. (2022) Investigation of OH-reactivity budget in the isoprene, α-pinene and m-xylene oxidation with OH under high NOx conditions, Atmospheric Environment, 271, 118916.
[https://doi.org/10.1016/j.atmosenv.2021.118916]
-
Samy, S., Zielinska, B. (2010) Secondary organic aerosol production from modern diesel engine emissions, Atmospheric Chemistry and Physics, 10, 609-625.
[https://doi.org/10.5194/acp-10-609-2010]
-
Sarrafzadeh, M., Wildt, J., Pullinen, I., Springer, M., Kleist, E., Tillmann, R., Schmitt, S.H., Wu, C., Mentel, T.F., Zhao, D., Hastie, D.R., Kiendler-Scharr, A. (2016) Impact of NOx and OH on secondary organic aerosol formation from β-pinene photooxidation, Atmospheric Chemistry and Physics, 16, 11237-11248.
[https://doi.org/10.5194/acp-16-11237-2016]
-
Sato, K., Fujitani, Y., Inomata, S., Morino, Y., Tanabe, K., Hikida, T., Shimono, A., Takami, A., Fushimi, A., Kondo, Y., Imamura, T., Tanimoto, H., Sugata, S. (2019) A study of volatility by composition, heating, and dilution measurements of secondary organic aerosol from 1,3,5-trimethylbenzene, Atmospheric Chemistry and Physics, 19, 14901-14915.
[https://doi.org/10.5194/acp-19-14901-2019]
-
Sato, K., Fujitani, Y., Inomata, S., Morino, Y., Tanabe, K., Ramasamy, S., Hikida, T., Shimono, A., Takami, A., Fushimi, A., Kondo, Y., Imamura, T., Tanimoto, H., Sugata, S. (2018) Studying volatility from composition, dilution, and heating measurements of secondary organic aerosols formed during α-pinene ozonolysis, Atmospheric Chemistry and Physics, 18, 5455-5466.
[https://doi.org/10.5194/acp-18-5455-2018]
-
Sato, K., Nakashima, Y., Morino, Y., Imamura, T., Kurokawa, J., Kajii, Y. (2017) Total OH reactivity measurements for the OH-initiated oxidation of aromatic hydrocarbons in the presence of NOx, Atmospheric Environment, 171, 272-278.
[https://doi.org/10.1016/j.atmosenv.2017.10.036]
-
Schlosser, E., Bohn, B., Brauers, T., Dorn, H.-P., Fuchs, H., Häseler, R., Hofzumahaus, A., Holland, F., Rohrer, F., Rupp, L.O., Siese, M., Tillmann, R., Wahner, A. (2007) Intercomparison of two hydroxyl radical measurement techniques at the atmosphere simulation chamber SAPHIR, Journal of Atmospheric Chemistry, 56, 187-205.
[https://doi.org/10.1007/s10874-006-9049-3]
-
Schnaiter, M., Järvinen, E., Vochezer, P., Abdelmonem, A., Wagner, R., Jourdan, O., Mioche, G., Shcherbakov, V.N., Schmitt, C.G., Tricoli, U., Ulanowski, Z., Heymsfield, A.J. (2016) Cloud chamber experiments on the origin of ice crystal complexity in cirrus clouds, Atmospheric Chemistry and Physics, 16, 5091-5110.
[https://doi.org/10.5194/acp-16-5091-2016]
-
Schneider, J., Höhler, K., Wagner, R., Saathoff, H., Schnaiter, M., Schorr, T., Steinke, I., Benz, S., Baumgartner, M., Rolf, C., Krämer, M., Leisner, T., Möhler, O. (2021) High homogeneous freezing onsets of sulfuric acid aerosol at cirrus temperatures, Atmospheric Chemistry and Physics, 21, 14403-14425.
[https://doi.org/10.5194/acp-21-14403-2021]
-
Sem, K., Jang, M., Pierce, R., Blum, P., Yu, Z. (2022) Characterization of atmospheric processes of brevetoxins in sea spray aerosols from red tide events, Environmental Science & Technology, 56, 1811-1819.
[https://doi.org/10.1021/acs.est.1c05740]
-
Seo, J., Lim, Y.B., Youn, D., Kim, J.Y., Jin, H.C. (2020) Synergistic enhancement of urban haze by nitrate uptake into transported hygroscopic particles in the Asian continental outflow, Atmospheric Chemistry and Physics, 20, 7575-7594.
[https://doi.org/10.5194/acp-20-7575-2020]
-
Shahpoury, P., Harner, T., Lammel, G., Lelieveld, S., Tong, H., Wilson, J. (2019) Development of an antioxidant assay to study oxidative potential of airborne particulate matter, Atmospheric Measurement Techniques, 12, 6529-6539.
[https://doi.org/10.5194/amt-12-6529-2019]
-
Shahpoury, P., Zhang, Z.W., Filippi, A., Hildmann, S., Lelieveld, S., Mashtakov, B., Patel, B.R., Traub, A., Umbrio, D., Wietzoreck, M., Wilson, J., Berkemeier, T., Celo, V., Dabek-Zlotorzynska, E., Evans, G., Harner, T., Kerman, K., Lammel, G., Noroozifar, M., Pöschl, U., Tong, H. (2022) Intercomparison of oxidative potential metrics for airborne particles identifies differences between acellular chemical assays, Atmospheric Pollution Research, 13, 101596.
[https://doi.org/10.1016/j.apr.2022.101596]
-
Shao, Y., Wang, Y., Du, M., Voliotis, A., Alfarra, M.R., O’Meara, S.P., Turner, S.F., McFiggans, G. (2022) Characterisation of the Manchester aerosol chamber facility, Atmospheric Measurement Techniques, 15, 539-559.
[https://doi.org/10.5194/amt-15-539-2022]
-
Shepson, P.B., Kleindienst, T.E., Edney, E.O., Namie, G.R., Pittman, J.H., Cupitt, L.T. (1985) Mutagenic activity of irradiated toluene nitrogen oxide (NOx)/water/air mixtures, Environmental Science & Technology, 19, 249-255.
[https://doi.org/10.1021/es00133a005]
-
Shepson, P.B., Kleindienst, T.E., Nero, C.M., Hodges, D.N., Cupitt, L.T., Claxton, L.D. (1987) Allyl chloride: the mutagenic activity of its photooxidation products, Environmental Science & Technology, 21, 568-573.
[https://doi.org/10.1021/es00160a007]
-
Shiraiwa, M., Ueda, K., Pozzer, A., Lammel, G., Kampf, C.J., Fushimi, A., Enami, S., Arangio, A.M., Fröhlich-Nowoisky, J., Fujitani, Y., Furuyama, A., Lakey, P.S.J., Lelieveld, J., Lucas, K., Morino, Y., Pöschl, U., Takahama, S., Takami, A., Tong, H., Weber, B., Yoshino, A., Sato, K. (2017) Aerosol health effects from molecular to global scales, Environmental Science & Technology, 51, 13545-13567.
[https://doi.org/10.1021/acs.est.7b04417]
-
Siese, M., Becker, K.H., Brockmann, K.J., Geiger, H., Hofzumahaus, A., Holland, F., Mihelcic, D., Wirtz, K. (2001) Direct measurement of OH radicals from ozonolysis of selected alkenes: a EUPHORE simulation chamber study, Environmental Science & Technology, 35, 4660-4667.
[https://doi.org/10.1021/es010150p]
-
Socorro, J., Lakey, P.S.J., Han, L., Berkemeier, T., Lammel, G., Zetzsch, C., Pöschl, U., Shiraiwa, M. (2017) Heterogeneous OH oxidation, shielding effects, and implications for the atmospheric fate of terbuthylazine and other pesticides, Environmental Science & Technology, 51, 13749-13754.
[https://doi.org/10.1021/acs.est.7b04307]
-
Song, M., Kim, M., Park, T., Lee, J.W., Lee, S.H., Bae, M.-S., Lee, T., Park, H.M. (2021) A review of large-scale smog chamber and research trends, Journal of the Korean Society for Environmental Technology, 22, 397-407, (in Korean with English abstract).
[https://doi.org/10.26511/JKSET.22.6.1]
-
Song, M., Zhang, C., Wu, H., Mu, J., Ma, Z., Liu, P., Liu, J., Zhang, Y., Chen, C., Fu, Y., Bi, X., Jiang, B., Mu, Y. (2018) The influence of UV-light irradiation and stable Criegee intermediate scavengers on secondary organic aerosol formation from isoprene ozonolysis, Atmospheric Environment, 191, 116-125.
[https://doi.org/10.1016/j.atmosenv.2018.08.014]
-
Srivastava, D., Vu, T.V., Tong, S., Shi, Z., Harrison, R.M. (2022) Formation of secondary organic aerosols from anthropogenic precursors in laboratory studies, npj Climate and Atmospheric Science, 5, 22.
[https://doi.org/10.1038/s41612-022-00238-6]
-
Steading, T.W. (1965) Environment simulation for studying the effects of air pollutants on computers, Journal of the Air Pollution Control Association, 15, 99-101.
[https://doi.org/10.1080/00022470.1965.10468349]
-
Steenhof, M., Gosens, I., Strak, M., Godri, K.J., Hoek, G., Cassee, F.R., Mudway, I.S., Kelly, F.J., Harrison, R.M., Lebret, E., Brunekreef, B., Janssen, N.A., Pieters, R.H. (2011) In vitro toxicity of particulate matter (PM) collected at different sites in the Netherlands is associated with PM composition, size fraction and oxidative potential-the RAPTES project, Particle and Fibre Toxicology, 8, 1-15.
[https://doi.org/10.1186/1743-8977-8-26]
-
Steinke, I., Funk, R., Busse, J., Iturri, A., Kirchen, S., Leue, M., Möhler, O., Schwartz, T., Schnaiter, M., Sierau, B., Toprak, E., Ullrich, R., Ulrich, A., Hoose, C., Leisner, T. (2016) Ice nucleation activity of agricultural soil dust aerosols from Mongolia, Argentina, and Germany, Journal of Geophysical Research: Atmospheres, 121, 13559-13576.
[https://doi.org/10.1002/2016JD025160]
-
Steinke, I., Hiranuma, N., Funk, R., Höhler, K., Tüllmann, N., Umo, N.S., Weidler, P.G., Möhler, O., Leisner, T. (2020) Complex plant-derived organic aerosol as ice-nucleating particles - more than the sums of their parts?, Atmospheric Chemistry and Physics, 20, 11387-11397.
[https://doi.org/10.5194/acp-20-11387-2020]
-
Stroud, C.A., Makar, P.A., Michelangeli, D.V., Mozurkewich, M., Hastie, D.R., Barbu, A., Humble, J. (2004) Simulating organic aerosol formation during the photooxidation of toluene/NOx mixtures: comparing the equilibrium and kinetic assumption, Environmental Science & Technology, 38, 1471-1479.
[https://doi.org/10.1021/es030546w]
-
Suda, S.R., Petters, M.D., Matsunaga, A., Sullivan, R.C., Ziemann, P.J., Kreidenweis, S.M. (2012) Hygroscopicity frequency distributions of secondary organic aerosols, Journal of Geophysical Research: Atmospheres, 117, D04207.
[https://doi.org/10.1029/2011JD016823]
-
Suda, S.R., Petters, M.D., Yeh, G.K., Strollo, C., Matsunaga, A., Faulhaber, A., Ziemann, P.J., Prenni, A.J., Carrico, C.M., Sullivan, R.C., Kreidenweis, S.M. (2014) Influence of functional groups on organic aerosol cloud condensation nucleus activity, Environmental Science & Technology, 48, 10182-10190.
[https://doi.org/10.1021/es502147y]
-
Svendby, T.M., Lazaridis, M., Tørseth, K. (2008) Temperature dependent secondary organic aerosol formation from terpenes and aromatics, Journal of Atmospheric Chemistry, 59, 25-46.
[https://doi.org/10.1007/s10874-007-9093-7]
-
Tajuelo, M., Rodríguez, A., Baeza-Romero, M.T., Aranda, A., Díazde-Mera, Y., Rodríguez, D. (2019) Secondary organic aerosol formation from α-methylstyrene atmospheric degradation: role of NO level, relative humidity and inorganic seed aerosol, Atmospheric Research, 230, 104631.
[https://doi.org/10.1016/j.atmosres.2019.104631]
-
Takekawa, H., Minoura, H., Yamazaki, S. (2003) Temperature dependence of secondary organic aerosol formation by photo-oxidation of hydrocarbons, Atmospheric Environment, 37, 3413-3424.
[https://doi.org/10.1016/S1352-2310(03)00359-5]
- Tang, X., Bi, M., Li, J., Zhang, X., Tang, D., Zhang, Y. (1982) Trial production and performance test of photochemical smog chamber, Environmental Chemistry, 1, 344-351, (in Chinese).
-
Tuet, W.Y., Chen, Y., Fok, S., Gao, D., Weber, R.J., Champion, J.A., Ng, N.L. (2017a) Chemical and cellular oxidant production induced by naphthalene secondary organic aerosol (SOA): effect of redox-active metals and photochemical aging, Scientific Reports, 7, 15157.
[https://doi.org/10.1038/s41598-017-15071-8]
-
Tuet, W.Y., Chen, Y., Xu, L., Fok, S., Gao, D., Weber, R.J., Ng, N.L. (2017b) Chemical oxidative potential of secondary organic aerosol (SOA) generated from the photooxidation of biogenic and anthropogenic volatile organic compounds, Atmospheric Chemistry and Physics, 17, 839-853.
[https://doi.org/10.5194/acp-17-839-2017]
-
Umo, N.S., Wagner, R., Ullrich, R., Kiselev, A., Saathoff, H., Weidler, P.G., Cziczo, D.J., Leisner, T., Möhler, O. (2019) Enhanced ice nucleation activity of coal fly ash aerosol particles initiated by ice-filled pores, Atmospheric Chemistry and Physics, 19, 8783-8800.
[https://doi.org/10.5194/acp-19-8783-2019]
-
VanReken, T.M. (2005) Cloud condensation nucleus activation properties of biogenic secondary organic aerosol, Atmospheric Chemistry and Physics, 110, D07206.
[https://doi.org/10.1029/2004JD005465]
-
Varutbangkul, V., Brechtel, F.J., Bahreini, R., Ng, N.L., Keywood, M.D., Kroll, J.H., Flagan, R.C., Seinfeld, J.H., Lee, A., Goldstein, A.H. (2006) Hygroscopicity of secondary organic aerosols formed by oxidation of cycloalkenes, monoterpenes, sesquiterpenes, and related compounds, Atmospheric Chemistry and Physics, 6, 2367-2388.
[https://doi.org/10.5194/acp-6-2367-2006]
-
Vera, T., Borás, E., Chen, J., Coscollá, C., Daële, V., Mellouki, A., Ródenas, M., Sidebottom, H., Sun, X., Yusá, V., Zhang, X., Muñoz, A. (2015) Atmospheric degradation of lindane and 1,3-dichloroacetone in the gas phase. Studies at the EUPHORE simulation chamber, Chemosphere, 138, 112-119.
[https://doi.org/10.1016/j.chemosphere.2015.05.061]
-
Wagner, R., Bunz, H., Linke, C., Möhler, O., Naumann, K.-H., Saathoff, H., Schnaiter, M., Schurath, U. (2006) Chamber simulations of cloud chemistry: the AIDA chamber, Environmental Simulation Chambers: Application to Atmospheric Chemical Processes, 67-82, Springer Netherlands.
[https://doi.org/10.1007/1-4020-4232-9_5]
-
Wagner, R., Möhler, O., Saathoff, H., Schnaiter, M., Skrotzki, J., Leisner, T., Wilson, T.W., Malkin, T.L., Murray, B.J. (2012) Ice cloud processing of ultra-viscous/glassy aerosol particles leads to enhanced ice nucleation ability, Atmospheric Chemistry and Physics, 12, 8589-8610.
[https://doi.org/10.5194/acp-12-8589-2012]
-
Wang, S., Tsona, N.T., Du, L. (2021) Effect of NOx on secondary organic aerosol formation from the photochemical transformation of allyl acetate, Atmospheric Environment, 255, 118426.
[https://doi.org/10.1016/j.atmosenv.2021.118426]
- Wang, W. (1998) Photochemical degradation of PAHs on smoke particles in atmosphere, Fuel and Energy Abstracts, 3(39), 223.
- Wang, W.X., Wang, D.H., Liu, Y.M., Ding, H.L., Zhang, G.M. (1989) The structure and characteristics of the evacuable photochemical smog chamber, Environmental Sciences, 9, 304-310, (in Chinese).
-
Wang, X., Liu, T., Bernard, F., Ding, X., Wen, S., Zhang, Y., Zhang, Z., He, Q., Lü, S., Chen, J., Saunders, S., Yu, J. (2014) Design and characterization of a smog chamber for studying gas-phase chemical mechanisms and aerosol formation, Atmospheric Measurement Techniques, 7, 301-313.
[https://doi.org/10.5194/amt-7-301-2014]
-
Wang, Y., Voliotis, A., Hu, D., Shao, Y., Du, M., Chen, Y., Kleinheins, J., Marcolli, C., Alfarra, M.R., McFiggans, G. (2022) On the evolution of sub- and super-saturated water uptake of secondary organic aerosol in chamber experiments from mixed precursors, Atmospheric Chemistry and Physics, 22, 4149-4166.
[https://doi.org/10.5194/acp-22-4149-2022]
-
Warren, B., Song, C., Cocker, D.R. (2008) Light intensity and light source influence on secondary organic aerosol formation for the m-xylene/NOx photooxidation system, Environmental Science & Technology, 42, 5461-5466.
[https://doi.org/10.1021/es702985n]
-
Weichenthal, S.A., Lavigne, E., Evans, G.J., Godri Pollitt, K.J., Burnett, R.T. (2016) Fine particulate matter and emergency room visits for respiratory illness. effect modification by oxidative potential, American Journal of Respiratory and Critical Care Medicine, 194, 577-586.
[https://doi.org/10.1164/rccm.201512-2434OC]
-
Wex, H., Petters, M.D., Carrico, C.M., Hallbauer, E., Massling, A., McMeeking, G.R., Poulain, L., Wu, Z., Kreidenweis, S.M., Stratmann, F. (2009) Towards closing the gap between hygroscopic growth and activation for secondary organic aerosol: Part 1 - Evidence from measurements, Atmospheric Chemistry and Physics, 9, 3987-3997.
[https://doi.org/10.5194/acp-9-3987-2009]
-
White, S., Angove, D., Azzi, M., Tibbett, A., Campbell, I., Patterson, M. (2015) An experimental investigation into the atmospheric degradation of piperazine, Atmospheric Environment, 108, 133-139.
[https://doi.org/10.1016/j.atmosenv.2015.02.063]
-
White, S., Angove, D., Li, K., Campbell, I., Element, A., Halliburton, B., Lavrencic, S., Cameron, D., Jamie, I., Azzi, M. (2018) Development of a new smog chamber for studying the impact of different UV lamps on SAPRC chemical mechanism predictions and aerosol formation, Environmental Chemistry, 15, 171.
[https://doi.org/10.1071/EN18005]
-
White, S.J., Azzi, M., Angove, D.E., Jamie, I.M. (2010) Modelling the photooxidation of ULP, E5 and E10 in the CSIRO smog chamber, Atmospheric Environment, 44, 5375-5382.
[https://doi.org/10.1016/j.atmosenv.2009.11.050]
-
Wilson Jr, W.E., Merryman, E.L., Levy, A., Taliaferro, H.R. (1971) Aerosol formation in photochemical smog: I. effect of stirring, Journal of the Air Pollution Control Association, 21, 128-132.
[https://doi.org/10.1080/00022470.1971.10469508]
- Wu, H., Mu, Y.J., Zhang, X.S. (2001) Rate constants for reactions of hydroxyl radicals with a series of alcohols by relative rate method, Acta Scientiae Circumstantiae, 21, 525-529, (in Chinese).
-
Wyche, K.P., Blake, R.S., Ellis, A.M., Monks, P.S., Brauers, T., Koppmann, R., Apel, E.C. (2007) Technical note: Performance of chemical ionization reaction time-of-flight mass spectrometry (CIR-TOF-MS) for the measurement of atmospherically significant oxygenated volatile organic compounds, Atmospheric Chemistry and Physics, 7, 609-620.
[https://doi.org/10.5194/acp-7-609-2007]
-
Xavier, C., Rusanen, A., Zhou, P., Dean, C., Pichelstorfer, L., Roldin, P., Boy, M. (2019) Aerosol mass yields of selected biogenic volatile organic compounds - a theoretical study with nearly explicit gas-phase chemistry, Atmospheric Chemistry and Physics, 19, 13741-13758.
[https://doi.org/10.5194/acp-19-13741-2019]
-
Yang, Z., Tsona, N.T., Li, J., Wang, S., Xu, L., You, B., Du, L. (2020) Effects of NO and SO2 on the secondary organic aerosol formation from the photooxidation of 1,3,5-trimethylbenzene: a new source of organosulfates, Environmental Pollution, 264, 114742.
[https://doi.org/10.1016/j.envpol.2020.114742]
-
Yee, L.D., Craven, J.S., Loza, C.L., Schilling, K.A., Ng, N.L., Canagaratna, M.R., Ziemann, P.J., Flagan, R.C., Seinfeld, J.H. (2013) Effect of chemical structure on secondary organic aerosol formation from n-alkanes, Atmospheric Chemistry and Physics, 13, 11121-11140.
[https://doi.org/10.5194/acp-13-11121-2013]
-
Yu, Z., Jang, M. (2018) Simulation of heterogeneous photooxidation of SO2 and NO2 in the presence of Gobi Desert dust particles under ambient sunlight, Atmospheric Chemistry and Physics, 18, 14609-14622.
[https://doi.org/10.5194/acp-18-14609-2018]
-
Yuan, C., Ma, Y., Diao, Y., Yao, L., Zhou, Y., Wang, X., Zheng, J. (2017) CCN activity of secondary aerosols from terpene ozonolysis under atmospheric relevant conditions, Journal of Geophysical Research: Atmospheres, 122(8), 4654-4669.
[https://doi.org/10.1002/2016JD026039]
-
Zádor, J., Turányi, T., Wirtz, K., Pilling, M.J. (2006) Measurement and investigation of chamber radical sources in the European Photoreactor (EUPHORE), Journal of Atmospheric Chemistry, 55, 147-166.
[https://doi.org/10.1007/s10874-006-9033-y]
-
Zhang, H., Li, H., Zhang, Y., Wang, X., Bi, F., Meng, L., Li, Y., Zhao, L., Zhang, X., Peng, Z., Mu, Y., Mellouki, W., Chai, F. (2023) Synergistic generation mechanisms of SOA and ozone from the photochemical oxidation of 1,3,5-trimethylbenzene: Influence of precursors ratio, temperature and radiation intensity, Atmospheric Research, 293, 106924.
[https://doi.org/10.1016/j.atmosres.2023.106924]
-
Zhang, J., Liu, D., Kong, S., Wu, Y., Li, S., Hu, D., Hu, K., Ding, S., Qiu, H., Li, W., Liu, Q. (2022) Contrasting resistance of polycyclic aromatic hydrocarbons to atmospheric oxidation influenced by burning conditions, Environmental Research, 211, 113107.
[https://doi.org/10.1016/j.envres.2022.113107]
-
Zhang, Q., Xu, Y., Jia, L. (2019) Secondary organic aerosol formation from OH-initiated oxidation of m-xylene: effects of relative humidity on yield and chemical composition, Atmospheric Chemistry and Physics, 19, 15007-15021.
[https://doi.org/10.5194/acp-19-15007-2019]
-
Zhang, R., Wang, G., Guo, S., Zamora, M.L., Ying, Q., Lin, Y., Wang, W., Hu, M., Wang, Y. (2015) Formation of urban fine particulate matter, Chemical Reviews, 115, 3803-3855.
[https://doi.org/10.1021/acs.chemrev.5b00067]
-
Zhang, X., Ortega, J., Huang, Y., Shertz, S., Tyndall, G.S., Orlando, J.J. (2018) A steady-state continuous flow chamber for the study of daytime and nighttime chemistry under atmospherically relevant NO levels, Atmospheric Measurement Techniques, 11, 2537-2551.
[https://doi.org/10.5194/amt-11-2537-2018]
-
Zhang, X., Schwantes, R.H., McVay, R.C., Lignell, H., Coggon, M.M., Flagan, R.C., Seinfeld, J.H. (2015) Vapor wall deposition in teflon chambers, Atmospheric Chemistry and Physics, 15, 4197-4214.
[https://doi.org/10.5194/acp-15-4197-2015]
-
Zhang, Z.-H., Hartner, E., Utinger, B., Gfeller, B., Paul, A., Sklorz, M., Czech, H., Yang, B.X., Su, X.Y., Jakobi, G., Orasche, J., Schnelle-Kreis, J., Jeong, S., Gröger, T., Pardo, M., Hohaus, T., Adam, T., Kiendler-Scharr, A., Rudich, Y., Zimmermann, R., Kalberer, M. (2022) Are reactive oxygen species (ROS) a suitable metric to predict toxicity of carbonaceous aerosol particles?, Atmospheric Chemistry and Physics, 22, 1793-1809.
[https://doi.org/10.5194/acp-22-1793-2022]
-
Zhao, D.F., Buchholz, A., Kortner, B., Schlag, P., Rubach, F., Fuchs, H., Kiendler-Scharr, A., Tillmann, R., Wahner, A., Watne, Å.K., Hallquist, M., Flores, J.M., Rudich, Y., Kristensen, K., Hansen, A.M.K., Glasius, M., Kourtchev, I., Kalberer, M., Mentel, Th.F. (2016) Cloud condensation nuclei activity, droplet growth kinetics, and hygroscopicity of biogenic and anthropogenic secondary organic aerosol (SOA), Atmospheric Chemistry and Physics, 16, 1105-1121.
[https://doi.org/10.5194/acp-16-1105-2016]
-
Zhao, D.F., Buchholz, A., Tillmann, R., Kleist, E., Wu, C., Rubach, F., Kiendler-Scharr, A., Rudich, Y., Wildt, J., Mentel, Th.F. (2017) Environmental conditions regulate the impact of plants on cloud formation, Nature Communications, 8, 14067.
[https://doi.org/10.1038/ncomms14067]
-
Zhao, Z., Le, C., Xu, Q., Peng, W., Jiang, H., Lin, Y.-H., Cocker, D.R., Zhang, H. (2019) Compositional evolution of secondary organic aerosol as temperature and relative humidity cycle in atmospherically relevant ranges, ACS Earth and Space Chemistry, 3, 2549-2558.
[https://doi.org/10.1021/acsearthspacechem.9b00232]
-
Zhou, S., Hwang, B.C.H., Lakey, P.S.J., Zuend, A., Abbatt, J.P.D., Shiraiwa, M. (2019) Multiphase reactivity of polycyclic aromatic hydrocarbons is driven by phase separation and diffusion limitations, Proceedings of the National Academy of Sciences of the United States of America, 116, 11658-11663.
[https://doi.org/10.1073/pnas.1902517116]
-
Zhou, Y., Zhang, H., Parikh, H.M., Chen, E.H., Rattanavaraha, W., Rosen, E.P., Wang, W., Kamens, R.M. (2011) Secondary organic aerosol formation from xylenes and mixtures of toluene and xylenes in an atmospheric urban hydrocarbon mixture: Water and particle seed effects (II), Atmospheric Environment, 45, 3882-3890.
[https://doi.org/10.1016/j.atmosenv.2010.12.048]
-
Zimmermann, K., Jariyasopit, N., Massey Simonich, S.L., Tao, S., Atkinson, R., Arey, J. (2013) Formation of nitro-PAHs from the heterogeneous reaction of ambient particle-bound PAHs with N2O5/NO3/NO2, Environmental Science & Technology, 47(15), 8434-8442.
[https://doi.org/10.1021/es401789x]
김준태 (한국과학기술연구원 지속가능환경연구단 선임연구원) (jt0102@kist.re.kr)
이지원 (한국과학기술연구원 지속가능환경연구단 선임연구원) (jiwonlee@kist.re.kr)
김경환 (한국과학기술연구원 지속가능환경연구단 선임연구원) (khkim@kist.re.kr)
김나진 (한국과학기술연구원 지속가능환경연구단 선임연구원) (njkim1012@kist.re.kr)
김지민 (한국과학기술연구원 지속가능환경연구단 학생연구원) (zeemin@kist.re.kr)
김 산 (한국과학기술연구원 지속가능환경연구단 학생연구원) (ykwonsan@kist.re.kr)
김환동 (한국과학기술연구원 지속가능환경연구단 학생연구원) (handon@kist.re.kr)
황민우 (한국과학기술연구원 지속가능환경연구단 학생연구원) (dkhg9379@u.sogang.ac.kr)
송예진 (한국과학기술연구원 지속가능환경연구단 학생연구원) (yjsong106@gmail.com)
김진영 (한국과학기술연구원 기후·환경연구소 소장) (jykim@kist.re.kr)
배귀남 (한국과학기술연구원 초미세먼지사업단 단장) (gnbae@kist.re.kr)
이승복 (한국과학기술연구원 지속가능환경연구단 책임연구원) (sblee2@kist.re.kr)