
판도라 측정 방법에 따른 서울 대류권 NO2 수직컬럼밀도의 일치성에 대한 연구
Abstract
We investigated the consistency of tropospheric nitrogen dioxide vertical column density (NO2 TropoVCD) depending on the different measurement methods (direct sun and MAX-DOAS mode) from Pandora in Seoul, Korea. The NO2 TropoVCD from both measurement methods converged within a narrow range (R=0.96), but the slope of the best-fit line was slightly higher than 1 indicating that a higher NO2 from direct sun, which is reliable, than MAX-DOAS mode. According to the dependence of the difference in measurement azimuth angles between the two modes, a tendency for the MAX-DOAS/direct sun ratio was decreased as it approached zero (the solar zenith angle decreased). The MAX-DOAS/direct sun ratio was linearly increased as the solar zenith angle increased (R=0.93). To understand the difference in measured zenith angle depending on methods, we investigated the NO2 vertical distribution retrieved from the MAX-DOAS method. The MAX-DOAS/direct sun ratio near sunrise (eastward) and sunset (westward), when the NO2 vertical gradient is pronounced, is close to 1. However, when the NO2 concentration vertical gradient is gradual around noon, the NO2 TropoVCD ratio is less than 1, suggesting that the influence of the NO2 concentration vertical gradient is not significant. Finally, to investigate the effect of aerosols, we examined the variation in MAX-DOAS/direct sun ratio depending on aerosol optical depth (AOD) measured by AERONET at the same location. Similar to changes in solar zenith angle, the MAX-DOAS/direct sun ratio increased as AOD increased. This AOD-dependent difference is considered to be more pronounced in MAX-DOAS methods that consider changes in climatological aerosols (O2-O2) compared to direct sun mode which estimates relatively accurate AOD. We expected that the differences in NO2 TropoVCD based on the Pandora measurement methods would not only contribute to more accurate satellite data validation but also facilitate improvements in satellite retrieval algorithms through the enhancement of NO2 vertical distribution accuracy.
Keywords:
Pandora, NO2 tropospheric vertical column density, Direct sun mode, MAX-DOAS mode1. 서 론
이산화질소 (Nitrogen dioxide, NO2)는 대류권에서 오존 생성 반응에 기여하는 기체상 대기오염물질로, 대기 화학에 있어서 매우 중요한 역할을 한다 (Crutzen, 1979). 장시간 NO2에 노출될 경우 천식 및 호흡기 관련 증상 등 인체에 부정적인 영향을 일으키는 것으로 알려져 있다 (Huangfu and Atkinson, 2020; Brunekreef et al., 2009). NO2는 번개, 토양, 생물성 연소 등 자연적으로 발생되기도 하지만, 자동차 그리고/또는 화석연료연소와 같은 인간 활동에 의해 주로 배 출된다 (Hudman et al., 2007; Zhang et al., 2003; Bond et al., 2001). 산업화, 경제성장 그리고 도시화로 대도시지역의 NO2 배출이 증가해 왔으며 (Zhang et al., 2007), 이에 따라 대도시의 높은 NO2 농도를 관리하고 감시하는 것이 중요시되어 왔다. NO2의 대기 중 체류시간은 다른 미량기체들에 비해 비교적 짧기 때문에 (Shah et al., 2020; Beirle et al., 2011), 배출원 및 기상조건에 의한 공간적 비균질성이 나타날 수 있다. 이러한 이유로 NO2 배출의 관리 감독을 위해 NO2의 시공간적인 분포를 이해하는 것은 매우 중요하다 (Lange et al., 2022).
NO2를 비롯한 대기오염물질들의 광역적 감시와 시공간적 분포를 파악하기 위해 저궤도 위성인 OMI (Ozone Monitoring Instrument)와 TROPOMI (TROPO spheric Monitoring Instrument) 그리고 우리나라 정지궤도 환경위성 GEMS (Geostationary Environmental Monitoring Spectrometer) 등 여러 위성을 이용한 관측이 활발히 수행되고 있다. 인공위성은 NO2의 대류권 수직컬럼밀도 (tropospheric vertical column density, TropoVCD)를 제공하지만, 복원 시 여러 가정에 의한 불확실성을 내포하고 있다. 따라서 판도라 (Pandora)와 다축 차등흡수분광법 (MAX-DOAS; Multi-AXis Differential Optical Absorption Spectroscopy)과 같이 시간해상도 및 복원 정확도가 높은 지상기반 원격측정 장비를 이용하여 인공위성에서 산출되는 NO2 TropoVCD 검증 및 정확도를 향상시키는 연구가 활발히 진행되고 있다 (Kim et al., 2023; Choi et al., 2021; Chan et al., 2019).
이 중 판도라는 대기질 감시 및 위성 검증 목적으로 2006년 NASA GSFC (Goddard Space Flight Center)에 서 처음 개발된 후 Pandonia Global Network (PGN; https://www.pandonia-global-network.org/)를 구축하여 전 세계 약 140여 개 주요 지점에서 NO2, 오존 그리고 포름알데하이드 등 다양한 미량기체들의 VCD를 상시 관측 중이다 (Cede, 2021). 우리나라에는 2012년 Distributed Regional Aerosol Gridded Observation Networks-North East Asia (DRAGON NE-Asia) 캠페인 기간에 서울특별시 연세대학교 등 국내 주요 지점에 판도라가 설치되어 (Chong et al., 2019), 현재까지 9지점에서 운영 중이다. 판도라는 일사가 있는 주간 구름이 없는 맑은 조건에서 두 가지 방식 (직달일사와 MAX-DOAS (산란일사) 방법)으로 측정을 수행한다. 관측된 원시자료는 DOAS 방법을 이용하여 경사컬럼밀도 (slant column density, SCD)를 산출한 후, 각 측정 방식의 복원 알고리즘을 통해 대기 중 미량기체들의 VCD를 산출한다 (Cede, 2021; Herman et al., 2009). 두 방식 모두 측정간격이 약 10분 이내인 높은 시간분해능을 가지기에 (Cede, 2021; Herman et al., 2019), 맑은 날 주간 동안 변화하는 주요 미량기체의 거동을 파악하기에 매우 용이하다.
두 가지 방법 중 직달일사 방법이 MAX-DOAS 방법에 비해 NO2 수직컬럼밀도를 측정하는 데 더 정확한 기술로 알려져 있다 (Herman et al., 2009; Cede et al., 2006; Brewer et al., 1973). MAX-DOAS 방법에 비해 직달일사 방법은 성층권과 대류권 NO2에 모두 민감하여, (1) 복잡한 복사전달모델 계산, (2) Ring 효과 고려, (3) NO2 연직분포에 대한 사전 지식, (4) 지표 반사도에 대한 정보, (5) 공간적 균질성을 가정할 필요가 없기 때문이다 (Herman et al., 2009; Cede et al., 2006). 하지만, MAX-DOAS 방법의 경우 앞서 언급한 조건에 대한 정확한 정보를 확보할 수 있다면, NO2 연직분포를 비롯하여 다양한 자료를 복원할 수 있는 장점이 있다 (Choi et al., 2021). 초기에는 직달일사로 산출되는 NO2 TropoVCD가 PGN에서 제공하는 공식 산출물 (official product)이었지만 최근 MAX-DOAS 방식으로 산출되는 NO2 TropoVCD 또한 공식 산출물로 제공되기에 두 산출물 간의 상세한 비교분석이 필요한 실정이다.
본 연구는 대표적인 도심지역인 서울특별시 연세대학교에서 운영 중인 판도라를 대상으로 두 가지 측정 방식에 따른 NO2 TropoVCD의 일치성을 분석하였다. 월평균 NO2 TropoVCD 변화를 통해 계절별 변동성 을 가진 NO2의 거동을 분석한 후, 두 측정 방식의 NO2 TropoVCD의 불일치성을 유발하는 원인을 조사하기 위해 측정 방위각, 천정각 그리고 NO2 연직분포 및 에어로졸의 영향을 차례로 살펴보았다.
2. 연구 방법
2. 1 측정장소 및 분석기간
본 연구는 서울특별시 서대문구에 위치한 연세대학교 (126.93°E, 37.56°N, 86 m)에서 운영 중인 판도라 자료를 사용하였다 (그림 1). 서울특별시는 2023년 기준으로 우리나라 인구 5,174만 명 중 약 19%인 977.6만 명이 거주하는 메가시티이다 (KOSIS, 2023). 또한 서울의 연간 질소산화물 (NOx) 배출량 62,575톤 중 도로이동오염원이 40.7%를 차지해 차량에 의한 NOx 배출이 주요한 지역이다 (NAIR, 2021). 분석기간은 2022년 1월부터 12월까지 1년간 측정된 자료를 분석하였다. 서울특별시 북서쪽에 위치한 연세대학교는 주변 여러 대학교들과 전철역 그리고 상업지역이 위치해 있어 유동인구가 매우 많고, 성산로 및 내부순환도로가 연세대 주변을 지나 차량에 의한 NOx 배출의 영향이 높은 지역이다.
2. 2 판도라
지상 원격관측 장비인 판도라는 0.6 nm의 분해능으로 자외선 및 가시광선 (280~525 nm)의 스펙트럼을 측정하여 대기 중 미량기체의 VCD를 산출하는 장비이다 (Herman et al., 2019). 또한 CCD (charge coupled device) 검출기가 포함된 분광계 (Avantes B.V.)는 온도조절 시스템을 이용하여 20°C로 유지되고 광학헤드 는 태양추적기, 시준기 (collimator), 렌즈로 구성되었으며 시야각 (Field of View)은 1.6°이다 (Herman et al., 2019; Tzortziou et al., 2012). 이러한 판도라 분광 시스템은 맑은 날 0.01 DU (1 DU=26.7 Pmolec. cm-2)의 매우 높은 정밀도를 가지며 신호 대 잡음비 (SNR) 역시 700 : 1로 높다.
판도라 관측은 두 가지 방식으로 수행된다. 직달 일사 방식 (NO2 Visible Synthetic reference version3, nvs3)은 천정각 (solar zenith angle)과 방위각 (azimuth angle)을 변화시키며 태양의 위치를 추적, 태양 직사광을 직접 측정하는 방식이다 (그림 2). MAX-DOAS (NO2 Visible Highest pointing zenith angle reference version3, nvh3)는 방위각을 정해진 각도로 고정시킨 채 천정각을 여러 각도 (pointing zenith angle; 0°, 60°, 75°, 89°)로 변화시키면서 관측하여 태양의 산란광을 측정하는 방식이다. 두 방식으로 측정된 원시자료는 BlickP 소프트웨어 복원알고리즘을 통해 DOAS 방법을 적용하여 SCD를 산출하고 NO2 VCD를 복원한다. 측정 방식에 따른 각기 다른 복원알고리즘이 적용되고 직달일사 방식 (rnvs3)은 총 수직컬럼밀도 (Total VCD=TropoVCD+성층권 VCD)를, MAX-DOAS (rnvh3) 방식은 NO2 TropoVCD를 산출한다 (Cede, 2021; Herman et al., 2009).
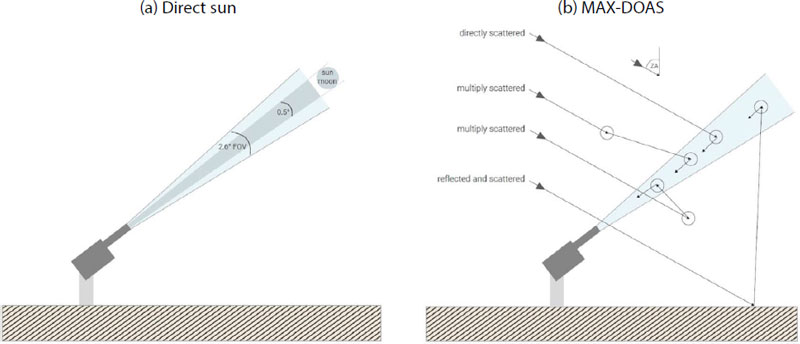
Pandora observation modes for (a) direct sun mode and (b) MAX-DOAS mode (Cede et al., 2023; Cede, 2021).
연세대학교 판도라는 PGN 표준 절차와 자료 처리에 따라 운영되고 있으며, 직달일사 방식은 태양직사광으로 산출된 SCD를 Air Mass Factor (AMF)로 보정함으로써, NO2 TotalVCD를 계산한다 (식 1).
(1) |
직달일사 방식으로 측정된 스펙트럼 복원 시 사용 한 파장범위는 400~470 nm이며, 복원에 사용한 미량기체 흡수단면적은 O3, NO2, O2O2, H2O, OIO, I2이다 (Cede et al., 2023; 표 1). 직달일사 방식의 NO2 TropoVCD는 NO2 TotalVCD에서 기후학적 (Climatologi-cal) 성층권 NO2 VCD를 제외하여 계산하였다 (Chong et al., 2019). 여기서 기후학적 성층권 NO2 VCD는 Odin 위성에 탑재된 OSIRIS (Optical Spectrograph and Infra-Red Imaging System)로 측정된 자료를 바탕으로 위도, 계절, 시간에 따른 함수로 고려된다 (Cede, 2021; Brohede et al., 2007).

Cross sections of considered trace gases in the spectral fitting used for Pandora retrieval program.
MAX-DOAS 방식의 측정 방위각은 160°로 남향을 관측한다 (그림 1). 일반적으로 MAX-DOAS는 복사전달모델로 계산된 NO2 연직분포 사전 지식 등과 측정된 ΔSCD (측정 SCD와 기준 SCD의 차이)를 이용하여 최적추정법 (optimal estimation)을 통해 복원되지만, PGN 산란일사 알고리즘은 식 (2)를 통해 NO2 TropoVCD로 산출된다.
(2) |
여기서 VCO2-O2는 O2-O2 기후학적 총 수직컬럼량 (total vertical column amount), ΔSC75°은 천정각이 75°인 미량기체 경사컬럼 (slant column)과 0° 기준컬럼의 차이를 의미하고, ΔSC75°,O2-O2와 ΔSC60°,O2-O2는 해당 천정각과 0°의 미량기체가 아닌 O2-O2의 차이를 뜻한다 (Cede, 2021). MAX-DOAS 방식으로 측정된 스펙트럼 복원 시 사용한 파장범위는 400~440 nm이며, 고려한 미량기체 흡수단면적은 직달일사의 알고리즘과 모두 동일하나, OIO와 I2는 사용되지 않는다 (Cede et al., 2023; 표 1). NO2 연직분포를 비롯한 PGN 자료 복원 방법은 Frieβ et al. (2019)과 Cede (2021)에 상세하게 기술되어 있다. 본 연구에서는 PGN에서 제공하는 판도라 v1.8 공식 산출물인 Level 2 (L2) 자료를 사용하였으며, 품질검증 (QA/QC)을 위하여 L2 자료품질표시 (data quality flags)가 0, 1, 10 그리고 11 (0=assured high quality, 1=assured medium quality, 10=not-assured high quality, 11=not-assured medium quality) 자료만 선별하여 분석하였다. 직달일사 방식과 MAX-DOAS 방식은 번갈아 가며 교차로 측정하기 때문에 두 방식의 측정시간 차이가 5분 이내인 자료를 선별하여 비교하였다. 분석 기간 동안 태양의 방위각과 천정각이 각각 MAX-DOAS 측정 방위각인 160°를 기준으로 ±2.5°, 최대 측정 천정각 60°를 기준으로 ±1.5° 이내 존재하는 자료 수를 조사한 결과, 전체 중 0.3%로 대부분의 MAX-DOAS 자료는 직달일사 영향이 없는 것으로 나타났다.
3. 결 과
3. 1 NO2 TropoVCD의 월변화
그림 3은 연구기간인 2022년 직달일사로 측정한 NO2 TropoVCD의 월변화이다. NO2 TropoVCD 전체 평균 (±표준편차)은 19.9±4.7 Pmolec. cm-2로 우리나라 주요 도심지역인 광주광역시 (9.99 Pmolec. cm-2), 부산광역시 (13.1 Pmolec. cm-2)보다 NO2 농도가 높았다 (Choi et al., 2023, 2021) (표 2). 이는 국외 도심지역인 그리스 아테네 (9.49 Pmolec. cm-2)와 중국 베이징 (11.05 Pmolec. cm-2)보다 높은 수준이었고, 일본 요코스카 (20.3 Pmolec. cm-2)와 유사한 농도 범위였다 (Drosoglou et al., 2023; Liu et al., 2023; Choi et al., 2021). 또한 이전 연구에서 보고된 서울의 2012~2017년 NO2 TotalVCD (32.3 Pmolec. cm-2)와 2015년 NO2 TropoVCD (40.1 Pmolec. cm-2)보다는 낮은 NO2 농도를 보여 (Chong et al., 2019; Herman et al. 2019), 최근 차량 배출을 비롯한 대기오염물질 관리정책이 효과적이었음을 짐작할 수 있다. 계절별로 살펴보면 겨울에 NO2 TropVCD가 가장 높았고 (26.2 Pmolec. cm-2), 뒤를 이어 봄 (19.4 Pmolec. cm-2), 가을 (18.5 Pmolec. cm-2) 그리고 여름 (15.5 Pmolec. cm-2) 순이었다. 이는 겨울철 인위적인 활동 (화석 연료 및 생물 연료 연소)에 의한 배출이 증가하는 동시에 일사량이 감소하여 광화학 반응이 억제되기 때문에 NO2의 대기 중 체류시간이 길어지기 때문이다. 반면, 여름에는 NO2의 빠른 광분해 속도 및 OH에 의한 효과적인 산화로 인해 NO2가 효율적으로 NO로 전환될 수 있기 때문이다 (Kanaya et al., 2014).
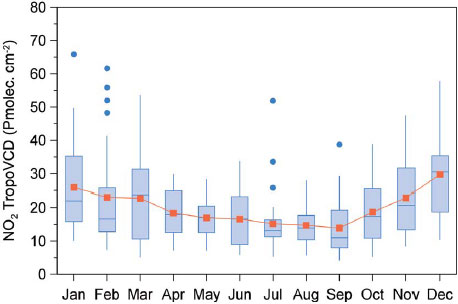
Monthly variation of NO2 TropoVCD from Pandora direct sun mode at Seoul in 2022. The blue circles and orange squares indicate the outlier and mean value of NO2 TropoVCD.
3. 2 측정 방식에 따른 NO2 TropoVCD 간의 상관관계
그림 4는 직달일사와 MAX-DOAS 방식으로 측정한 NO2 TropoVCD 분포도이다. 앞서 언급하였듯이 두 측정 방식은 교차로 측정하기 때문에 측정시간 차이가 5분 이내의 자료를 선정하여 비교하였다. 직달일사와 MAX-DOAS 방식의 NO2 TropoVCD의 평균 과 표준편차는 17.0±10.6 Pmolec. cm-2와 15.6±9.8 Pmolec. cm-2로 직달일사 측정 방식이 MAX-DOAS 방식 대비 평균 약 9.0% 높았다. 측정 방법에 따른 NO2 TropoVCD의 상관계수 (R)는 0.96으로 상관성이 매우 높았으며 유의확률도 매우 작았지만 (p<001), 최적합치선의 기울기는 1.04로 직달일사 방식으로 측정한 NO2 TropoVCD 값이 MAX-DOAS 방식보다 다소 높은 경향을 보였다. 계절별로 살펴보면, 모든 계절에서 좋은 상관성 (R≥0.94)을 보였으나, 기울기는 약간의 차이를 보였다. 여름의 최적합치선 기울기는 0.96으로 가장 편차가 작았으며, 뒤를 이어 겨울 (0.87), 가을 (0.86), 봄 (0.85) 순으로, 여름을 제외하고는 대부분 유사한 범위에 수렴하였다.
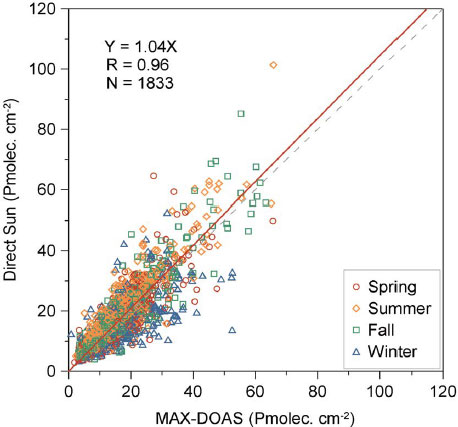
Scatter plot of NO2 TropoVCD between Pandora direct sun mode and MAX-DOAS mode depending on the season. The red line and gray dashed line indicate best-fit curve and 1 : 1 lines, respectively.
결과적으로 판도라의 두 가지 측정 방식으로 산출된 NO2 TropoVCD는 높은 상관성이 있음에도 불구하고, 직달일사의 NO2 TropoVCD가 다소 높은 것을 확인하였다. 이러한 NO2 TropoVCD의 불일치를 유발하는 원인으로는 (1) 측정 방식에 따라 각기 다른 방위각을 관측하거나 (수평적 NO2 비균질성), (2) 시간에 따른 측정 천정각의 차이가 있거나 (수직적 NO2 비균질성), (3) 대기 중 에어로졸의 영향 때문으로 생각되어 추가 분석을 실시하였다.
3. 3 방위각 차이에 따른 NO2 TropoVCD의 비율 변화
판도라의 측정 방식에 따른 방위각 차이 영향을 살펴보기 위해 직달일사 방식의 태양 방위각과 MAX-DOAS 방식의 측정 방위각의 차이 (Δ방위각; ΔAzimuth angle)를 통해 NO2 TropoVCD의 비율 (MAX-DOAS/direct Sun)을 비교 분석하였다 (그림 5). 즉, Δ방위각이 0°이면, 태양의 방위각과 MAX-DOAS 측정 방위각이 남향으로 같음을 의미하며 (측정 방위각은 160°), 수평적 NO2 비균질성 영향은 비교적 최소화된 측정조건을 의미한다.
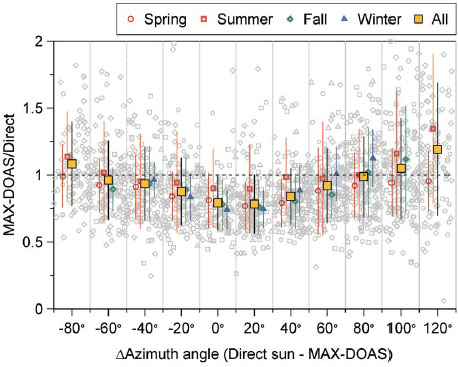
The mean and standard deviation of the NO2 TropoVCD ratio (MAX-DOAS/direct sun) depending on the difference in azimuth angle between the direct sun and MAXDOAS mode (ΔAzimuth angle: direct sun - MAX-DOAS). Filled and open symbols indicate the overall and seasonal mean of the NO2 TropoVCD ratio depending on ΔAzimuth angle. The dashed horizontal line represents unity of NO2 TropoVCD ratio.
Δ방위각 구간별 전체 평균을 살펴보면 NO2 TropoVCD의 비가 20°에서 0.78로 가장 낮아 Δ방위각이 0°에 근접할수록 (남향일수록) 직달일사 NO2가 더 높은 특성을 보였다. 계절별로 살펴보면, Δ방위각이 20°일 때 (겨울은 0°), NO2 TropoVCD의 비는 겨울에 0.74로 가장 낮았고, 뒤를 이어 가을 (0.76), 봄 (0.77) 순이었으며, 여름은 NO2 TropoVCD의 비가 0.90으로 최소값을 보였다. 반대로 Δ방위각의 절대값이 증가할수록 (일출인 동향 (-) 혹은 일몰인 서향 (+)), NO2 TropoVCD의 비가 1에 근접하거나 높아 MAX-DOAS의 농도가 직달일사에 비해 높았으며, 전체 평균 기준으로 120°에서 1.19로 가장 높았다. 이는 수평적 NO2 비균질성에 의한 영향일 수 있지만, 복원 방법 차이에 기인한 영향을 배제할 수 없다. 계절별로 봄철은 동향인 -80°에서 0.99로 가장 높았지만, 나머지 계절은 서향 (여름: 117.5°, 가을: 102.5°, 겨울: 85°)에서 비가 1.13에서 1.35로 MAX-DOAS가 높았다. 즉, 정리하자면, Δ방위각의 절대값이 크고 천정각이 높은 일출 (동향) 혹은 일몰 (서향) 조건에서는 MAX-DOAS와 직달일사의 차이가 작았지만, Δ방위각이 작고 천정각이 비교적 낮은 남향의 경우 그 차이가 증가하는 것을 확인할 수 있었다.
3. 4 태양의 천정각 구간별 NO2 TropoVCD의 비율 변화
태양의 천정각에 따라 차이가 유발되는지 확인하기 위하여, 천정각 구간별 NO2 TropoVCD 비의 변화를 살펴보았다 (그림 6). 천정각 구간별 NO2 TropoVCD의 비를 살펴보면, 천정각이 높아질수록 NO2 TropoVCD의 비율이 점점 증가하는 선형관계를 보여 (R=0.93), 앞선 Δ방위각 결과와 마찬가지로 일몰 혹은 일출시간에 MAX-DOAS 방식이 직달일사 NO2 TropoVCD에 근접하는 것을 확인하였다. 전체 평균을 살펴보면, 천정각이 20°일 때, NO2 TropoVCD의 비가 0.88로 MAX-DOAS 방식이 직달일사에 비해 낮았지만, 천정각이 80°로 높아지면 비가 1.07로 MAX-DOAS 방식이 좀 더 높았다. 계절별로 살펴보면, 모든 계절 천정각 증가에 따른 비율증가 추세는 동일하였으나, 여름철은 다른 계절에 비해 비율 자체가 높아 (0.95~1.3), 천정각이 낮은 조건에서도 두 측정 방식에 따른 편차가 가장 작았다. 이는 태양을 추적하는 직달일사 방식은 낮은 천정각으로 측정하는 반면, MAX-DOAS 방식은 천정각 60° 이상만 측정하기에 NO2 연직 농도 구배가 급격할 시 (예를 들어, 다량의 NO2가 상층 부근에 존재할 경우), 두 측정 방식 간의 NO2 TropoVCD 편차가 발생할 가능성이 있기에 NO2 연직분포를 살펴보았다.
3. 5 NO2 연직분포 및 에어로졸의 영향
다음은 MAX-DOAS 방식으로 측정한 계절별 NO2 TropoVCD의 연직분포이다 (그림 7). 고도별 해상도는 약 0.2 km이며, 약 4 km까지의 연직분포를 제공하지만 농도수준이 낮아, 본 연구에서는 고도 3 km까지만 분석에 사용하였다. 전체적인 연직분포를 살펴보았을 때, 대부분의 고농도 NO2 TropoVCD는 지표 부근이 아닌 고도 ~1 km 부근 혹은 그 이하에 주로 존재하는 것을 확인하였다. 계절별로 살펴보았을 경우, 봄철은 오전에 ~1 km 부근에서 고농도를 보이다 출근시간인 7~8시에는 차량 통행에 의해서 고농도 NO2의 고도가 약 0.6~0.8 km로 감소하였다. 이후 일사량이 증가함에 따라 15~16시에 고농도 고도가 다시 ~1 km로 상승하였다. 이러한 결과는 한미 대기질 합동연구 (KORUS-AQ) 기간 동안 서울 올림픽 공원에서 DC-8 항공기로 수행된 NO2 연직분포 실측 결과와 유사하였다 (Crawford et al., 2021). 오전에는 고도 500 m 이하에서 대부분의 배출된 NO2가 존재하지만 오후로 접어들면서 경계층이 높아짐에 따라 배출되는 NO2가 더 높은 고도로 혼합된다. 이후 지속적인 NOx 배출과 짧은 수명으로 인해 경계층 내에서 고도가 상승할수록 농도는 감소하였다.
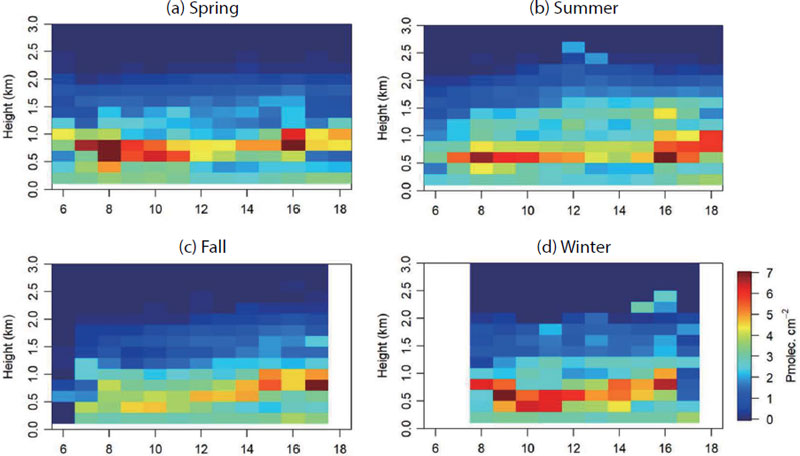
The diurnal variation of NO2 vertical profile below 3 km altitude from Pandora MAX-DOAS mode. (a) spring, (b) summer, (c) fall, (d) winter.
여름철 역시 봄철과 유사하게, 오전에 고농도를 보이고, 오후에 비교적 높은 고도에서 고농도 NO2를 보였지만, 고농도 고도가 0.6 km로 봄에 비해 낮았다. 겨울철도 마찬가지로 오전에 고농도를 약 0.8 km에서 보이다, 10~11시경 고농도 고도가 0.4 km까지 감소한 후, 다시 급격하게 상승하는 특성을 보였다. 대체적으로 천정각이 낮은 정오 시간대에 NO2 농도구배가 일출/일몰시간에 비해 완만하며, 고농도 고도 역시 낮아지는 것을 확인하였다. 측정 방법에 따른 NO2 TropoVCD 비와 함께 살펴본다면, NO2의 농도구배가 뚜렷한 일출과 일몰 부근 시간은 NO2 TropoVCD 비가 1에 가까운 반면, 상대적으로 NO2 농도 구배가 완만한 정오 부근은 NO2 TropoVCD 비가 1보다 작았다. 따라서 앞서 언급한 다량의 NO2가 상층에 존재하여, 측정 천정각 차이로 인해 유발되는 영향은 크지 않은 것으로 생각된다.
다음으로 에어로졸 광학적 깊이 (aerosol optical depth; AOD)가 NO2 TropoVCD 비에 미치는 영향을 알아보기 위해 측정장소와 동일한 지점 (Yonsei_University)에서 측정된 AERONET (AErosol RObotic NETwork) 자료를 이용하였다. 판도라에서도 직달일사 방식에서 AOD를 제공하지만, 본 연구에서는 많은 연구에서 검증된 AERONET 440 nm 파장 자료를 이용하였다. 시간평균 AOD 구간에 따른 MAX-DOAS와 직달일사 NO2 TropoVCD 비를 살펴보면 (그림 8), 앞선 천정각 변화와 유사하게 AOD가 높아질수록 MAX-DOAS와 직달일사의 비가 증가하는 것을 확인하였다. 즉, 컬럼 에어로졸의 양이 적은 조건에서는 NO2 TropoVCD 비가 0.77로 MAX-DOAS의 저평가가 두드러지지만, 관측기간 서울 AOD 평균값 (0.41) 부근인 0.4인 조건에 가까워지면, 비가 0.95로 1에 가까워지고, 오염된 조건 (80퍼센타일) AOD (0.61)에 가까운 경우 1.03으로 1을 다소 상회하였다. 이후 오염이 심한 조건 (99퍼센타일) AOD인 1.5부근까지 두 방법에 따른 비가 1.07까지 상승하였다.
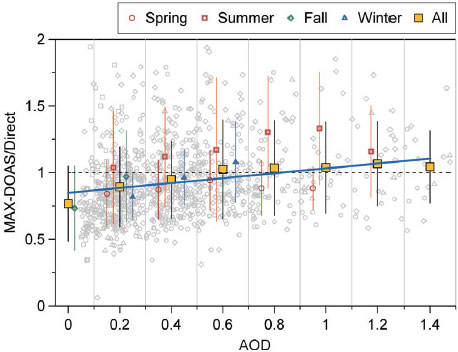
Same as Fig. 5 except for the aerosol optical depth (AOD) at 440 nm from AERONET (Yonsei_University).
Kanaya et al. (2014)은 OMI 위성과 MAX-DOAS NO2 비를 조사한 결과, 본 연구 결과와 유사하게 AOD에 따른 NO2 비의 변화를 밝혔다. 이러한 편차는 위성 자료 복원 시 NO2 연직분포 및 에어로졸/구름의 영향이 고려되지만 (Bucsela et al., 2013; Boersma et al., 2011), 최적 추정 (optimal estimation) 알고리즘을 사용하는 MAX-DOAS에 비해 부정확한 가정이 일부 영향으로 알려져 있다 (Ma et al., 2013; Shaiganfar et al., 2011; Leitão et al., 2010). 유사한 맥락으로 판도라 측정 방식에 따른 에어로졸 복원 방법을 살펴보면, 직달일사 방식은 AOD를 직접 복원하여 비교적 정확한 영향을 고려하는 반면, MAX-DOAS 방식은 기후학적인 에어로졸 (O2-O2)의 변화를 고려하기 때문에 직달일사와 차이가 있다. 따라서 직달일사 방식으로 복원된 AOD가 비교적 정확하게 에어로졸 영향을 대부분 제거하였다고 생각한다면, 에어로졸 조건에서는 상대적으로 부정확한 MAX-DOAS 방식의 에어로졸 (O2-O2)의 영향으로 NO2 복원에 불확실성이 커진 것으로 생각된다.
4. 결 론
본 연구는 서울 연세대학교에서 운영 중인 판도라를 대상으로 Pandora Global Network (PGN)에서 공식산출물로 제공하는 직달일사 방식과 최근 제공하기 시작한 MAX-DOAS 방식으로 산출한 NO2 TropoVCD의 일치성에 대해 조사하였다. 태양을 추적하며 측정하는 직달일사 방식과 방위각을 고정시킨 채 정해진 여러 천정각으로 측정하는 MAX-DOAS 방식은 측정 방식뿐만 아니라 자료 복원 및 처리 과정이 상이하여 NO2 TropoVCD의 편차가 발생할 수 있어 이에 대한 연구가 필요하다. 판도라 직달일사로 측정한 2022년 평균 NO2 TropoVCD는 19.9±4.7 Pmolec. cm-2로 선행연구에서 보고된 다른 도심지역 농도 수준을 나타내었다. 계절별 배출원 영향 및 기상조건 에 따른 NO2 체류시간의 영향으로 겨울철에 가장 높았고, 여름에 농도가 가장 낮았다. 직달일사와 MAX-DOAS 방식으로 5분 이내에 측정된 NO2 TropoVCD의 상관성은 매우 높았지만 (R=0.96), 평균 농도는 각각 17.0±10.6 Pmolec. cm-2와 15.6±9.8 Pmolec. cm-2로 직달일사 방식이 MAX-DOAS 방식보다 약 9.0% 높았다. 모든 계절에 걸쳐 상관성이 좋았지만 (R≥ 0.94), 여름의 최적합치선 기울기가 0.96으로 가장 편차가 작았다. 두 측정 방식 간 방위각 차이 (Δ방위각)에 따른 NO2 TropoVCD의 편차를 조사한 결과, Δ방위각이 0°에 수렴할수록 (천정각이 낮은 남향), 직달 일사 방식의 NO2 TropoVCD가 MAX-DOAS 방식보 다 더 커지는 특성을 보였으며 Δ방위각이 클수록 (천정각이 높은 동향 혹은 서향), 두 방식의 NO2 TropoVCD가 유사하였다. 또한 태양의 천정각이 높아질수록 MAX-DOAS/직달일사 비율이 1 이하에서 점점 증가하는 선형관계를 보였으나, 여름철에는 천정각이 낮은 조건에서도 두 측정 방식의 편차가 작은 결과를 보였다. 측정 방식에 따른 측정 천정각의 차이 영향인지 파악하기 위해, MAX-DOAS 방식으로 복원된 NO2 연직분포를 살펴보았다. NO2 연직 농도구배가 뚜렷한 일출 (동향)과 일몰 (서향) 부근의 MAX-DOAS/직달일사 비율은 1에 가까운 반면, NO2 연직 농도구배가 완만한 정오의 NO2 TropoVCD 비는 1보다 작아 NO2의 연직 농도구배의 영향은 크지 않은 것으로 생각된다. 마지막으로 에어로졸의 효과를 살펴보기 위하여 동일 지점에서 AERONET으로 측정된 에어로졸 광학적 깊이 (AOD)에 따른 변화를 살펴본 결과, 천정각 변화와 유사하게 AOD가 높아질수록 MAX-DOAS/직달일사 비율이 증가하였다. 이러한 AOD에 의존적인 차이는 비교적 정확한 AOD를 복원하는 직달일사 방식에 비해, 기후학적인 에어로졸 (O2-O2)의 변화를 고려하는 MAX-DOAS 방식의 상대적으로 부정확한 에어로졸 복원이 영향을 끼친 것으로 생각된다. 본 연구를 통해 조사된 판도라 측정 방법에 따른 NO2 TropoVCD 차이는 비교적 정확한 직달일사 방법 대비 MAX-DOAS 방법에 대한 편차 정도 및 유발 인자에 대한 정보를 제공함으로써, 향후 보다 정확한 인공위성자료 검증뿐만 아니라, 정확도가 향상된 NO2 연직분포를 통해 인공위성 복원알고리즘 개선 등을 도모할 수 있을 것으로 기대한다.
Acknowledgments
이 연구는 한국연구재단 기본연구 (NRF-2022R1F1A1063339)와 2023학년도 한국외국어대학교 교원연구지원사업 지원으로 수행되었습니다. 판도라 기기 운영 및 자료를 제공해 주신 연세대학교 김준 교수님께 감사드립니다.
References
-
Beirle, S., Boersma, K.F., Platt, U., Lawrence, M.G., Wagner, T. (2011) Megacity emissions and lifetimes of nitrogen oxides probed from space, Science, 333(6050), 1737-1739.
[https://doi.org/10.1126/science.1207824]
-
Boersma, K.F., Eskes, H.J., Dirksen, R.J., van der A, R.J., Veefkind, J.P., Stammes, P., Huijnen, V., Kleipool, Q.L., Sneep, M., Claas, J., Leitão, J., Richter, A., Zhou, Y., Brunner, D. (2011) An improved tropospheric NO2 column retrieval algorithm for the Ozone Monitoring Instrument, Atmospheric Measurement Techniques, 4, 1905-1928.
[https://doi.org/10.5194/amt-4-1905-2011]
-
Bond, D.W., Zhang, R., Tie, X., Brasseur, G., Huffines, G., Orville, R.E., Boccippio, D.J. (2001) NOx production by lightning over the continental United States, Journal of Geophysical Research: Atmospheres, 106(D21), 27701-27710.
[https://doi.org/10.1029/2000JD000191]
- Brewer, A.W., McElroy, C.T., Kerr, J.B. (1973) Nitrogen Dioxide Concentrations in the Atmosphere, Nature, 246, 129-133.
- Brohede, S.M., Haley, C.S., McLinden, C.A., Sioris, C.E., Murtagh, D.P., Petelina, S.V., Llewellyn, E.J., Bazureau, A., Goutail, F., Randall, C.E., Lumpe, J.D., Taha, G., Thomasson, L.W., Gordley, L.L. (2007) Validation of Odin/OSIRIS stratospheric NO2 profiles, Journal of Geophysical Research: Atmospheres, 112.
- Brunekreef, B., Beelen, R.M.J., Hoek, G., Schouten, L.J., Bausch- Goldbohm, S., Fischer, P., Armstrong, B., Hughes, E., Jerrett, M. (2009) Effects of long-term exposure to traffic-related air pollution on respiratory and cardiovascular mortality in the Netherlands: the NLCS-AIR study, Research report (Health Effects Institute), 139, 5-71.
-
Bucsela, E.J., Krotkov, N.A., Celarier, E.A., Lamsal, L.N., Swartz, W.H., Bhartia, P.K., Boersma, K.F., Veefkind, J.P., Gleason, J.F., Pickering, K.E. (2013) A new stratospheric and tropospheric NO2 retrieval algorithm for nadir-viewing satellite instruments: applications to OMI, Atmospheric Measurement Techniques, 6, 2607-2626.
[https://doi.org/10.5194/amt-6-2607-2013]
- Cede, A. (2021) Manual for Blick software suite 1.8, 10 Sep 2021, Issue 1.8-4, available at: https://www.pandonia-global-network.org/wp-content/uploads/2021/09/Blick-SoftwareSuite_Manual_v1-8-4.pdf, (accessed on Jan. 12, 2024).
- Cede, A., Herman, J., Richter, A., Krotkov, N., Burrows, J. (2006) Measurements of nitrogen dioxide total column amounts using a Brewer double spectrophotometer in direct Sun mode, Journal of Geophysical Research: Atmospheres, 111(D5).
- Cede, A., Tiefengraber, M., Gebetsberger, M., Spinei Lind, E. (2023) Pandonia Global Network Data Products Readme Document, Tech. rep., PGN-DataProducts-Readme, version 1.8-8, 31 November 2023. https://www.pandonia-global-network.org/wp-content/uploads/2023/11/PGN_DataProducts_Readme_v1-8-8.pdf, (last access: Jan. 12, 2024).
-
Chan, K.L., Wang, Z., Ding, A., Heue, K.P., Shen, Y., Wang, J., Zhang, F., Shi, Y., Hao, N., Wenig, M. (2019) MAX-DOAS measurements of tropospheric NO2 and HCHO in Nanjing and a comparison to ozone monitoring instrument observations, Atmospheric Chemistry and Physics, 19(15), 10051-10071.
[https://doi.org/10.5194/acp-19-10051-2019]
-
Choi, Y., Kanaya, Y., Takashima, H., Irie, H., Park, K., Chong, J. (2021) Long-Term Variation in the Tropospheric Nitrogen Dioxide Vertical Column Density over Korea and Japan from the MAX-DOAS Network, 2007-2017, Remote Sensing, 13(10), 1937.
[https://doi.org/10.3390/rs13101937]
-
Choi, Y., Kanaya, Y., Takashima, H., Park, K., Lee, H., Chong, J., Kim, J.H., Park, J.S. (2023) Changes in Tropospheric Nitrogen Dioxide Vertical Column Densities over Japan and Korea during the COVID-19 Using Pandora and MAXDOAS, Aerosol and Air Quality Research, 23, 220145.
[https://doi.org/10.4209/aaqr.220145]
-
Chong, H., Lee, H., Koo, J.-H., Kim, J., Jeong, U., Kim, W., Kim, S.-W., Herman, J.R., Abuhassan, N.K., Ahn, J.-Y., Park, J.-H., Kim, S.-K., Moon, K.-J., Choi, W.-J., Park, S.S. (2019) Regional Characteristics of NO2 Column Densities from Pandora Observations during the MAPS-Seoul Campaign, Aerosol and Air Quality Research, 18(9), 2207-2219.
[https://doi.org/10.4209/aaqr.2017.09.0341]
- Crawford, J.H., Ahn, J.-Y., Al-Saadi, J., Chang, L., Emmons, L.K., Kim, J., Lee, G., Park, J.-H., Park, R.J., Woo, J.H., Song, C.-K., Hong, J.-H., Hong, Y.-D., Lefer, B.L., Lee, M., Lee, T., Kim, S., Min, K.-E., Yum, S.S., Shin, H.J., Kim, Y.-W., Choi, J.-S., Park, J.-S., Szykman, J.J., Long, R.W., Jordan, C.E., Simpson, I.J., Fried, A., Dibb, J.E., Cho, S., Kim, Y.P. (2021) The Korea-United States Air Quality (KORUSAQ) field study, Elementa: Science of the Anthropocene, 9.
- Crutzen, P.J. (1979) The role of NO and NO2 in the chemistry of the troposphere and stratosphere, Annual Review of Earth and Planetary Sciences, 7(1), 443-472.
-
Drosoglou, T., Koukouli, M.E., Raptis, I.P., Kazadzis, S., Pseftogkas, A., Eleftheratos, K., Zerefos, C. (2023) Nitrogen dioxide spatiotemporal variations in the complex urban environment of Athens, Greece, Atmospheric Environment, 314, 120115.
[https://doi.org/10.1016/j.atmosenv.2023.120115]
- Frieß, U., Beirle, S., Alvarado Bonilla, L., Bösch, T., Friedrich, M.M., Hendrick, F., Piters, A., Richter, A., van Roozendael, M., Rozanov, V.V., Spinei, E., Tirpitz, J.L., Vlemmix, T., Wagner, T., Wang, Y. (2019) Intercomparison of MAX-DOAS vertical profile retrieval algorithms: studies using synthetic data, Atmospheric Measurement Techniques, 12, 2155-2181.
-
Herman, J., Abuhassan, N., Kim, J., Kim, J., Dubey, M., Raponi, M., Tzortziou, M. (2019) Underestimation of column NO2 amounts from the OMI satellite compared to diurnally varying ground-based retrievals from multiple PANDORA spectrometer instruments, Atmospheric Measurement Techniques, 12(10), 5593-5612.
[https://doi.org/10.5194/amt-12-5593-2019]
-
Herman, J., Cede, A., Spinei, E., Mount, G., Tzortziou, M., Abuhas san, N. (2009) NO2 column amounts from groundbased Pandora and MFDOAS spectrometers using the direct-sun DOAS technique: Intercomparisons and application to OMI validation, Journal of Geophysical Research: Atmospheres, 114(D13).
[https://doi.org/10.1029/2009JD011848]
-
Huangfu, P., Atkinson, R. (2020) Long-term exposure to NO2 and O3 and all-cause and respiratory mortality: A systematic review and meta-analysis, Environment International, 144, 105998.
[https://doi.org/10.1016/j.envint.2020.105998]
-
Hudman, R.C., Jacob, D.J., Turquety, S., Leibensperger, E.M., Murray, L.T., Wu, S., Gilliland, A., Avery, M., Bertram, T.H., Brune, W. (2007) Surface and lightning sources of nitrogen oxides over the United States: Magnitudes, chemical evolution, and outflow, Journal of Geophysical Research: Atmospheres, 112(D12).
[https://doi.org/10.1029/2006JD007912]
- Kanaya, Y., Irie, H., Takashima, H., Iwabuchi, H., Akimoto, H., Sudo, K., Gu, M., Chong, J., Kim, Y.J., Lee, H., Li, A., Si, F., Xu, J., Xie, P.-H., Liu, W.-Q., Dzhola, A., Postylyakov, O., Ivanov, V., Grechko, E., Terpugova, S., Panchenko, M. (2014) Long-term MAX-DOAS network observations of NO2 in Russia and Asia (MADRAS) during the period 2007-2012: instrumentation, elucidation of climatology, and comparisons with OMI satellite observations and global model simulations, Atmospheric Chemistry and Physics, 14(15), 7909-7927.
-
Kim, S., Kim, D., Hong, H., Chang, L.-S., Lee, H., Kim, D.-R., Kim, D., Yu, J.-A., Lee, D., Jeong, U. (2023) First-time comparison between NO2 vertical columns from Geostationary Environmental Monitoring Spectrometer (GEMS) and Pandora measurements, Atmospheric Measurement Techniques, 16(16), 3959-3972.
[https://doi.org/10.5194/amt-16-3959-2023]
- Korean Statistical Information Service (KOSIS) Ministry of the Interior and Safety (2023) Population Statistics Based on Resident Registration. https://kosis.kr/statHtml/statHtml.do?orgId=101&tblId=DT_1B040A3&checkFlag=N
-
Lange, K., Richter, A., Burrows, J.P. (2022) Variability of nitrogen oxide emission fluxes and lifetimes estimated from Sentinel-5P TROPOMI observations, Atmospheric Chemistry and Physics, 22(4), 2745-2767.
[https://doi.org/10.5194/acp-22-2745-2022]
-
Leitão, J., Richter, A., Vrekoussis, M., Kokhanovsky, A., Zhang, Q.J., Beekmann, M., Burrows, J.P. (2010) On the improvement of NO2 satellite retrievals - aerosol impact on the airmass factors, Atmospheric Measurement Techniques, 3, 475-493.
[https://doi.org/10.5194/amt-3-475-2010]
-
Liu, O., Li, Z., Lin, Y., Fan, C., Zhang, Y., Li, K., Zhang, P., Wei, Y., Chen, T., Dong, J., de Leeuw, G. (2023) Evaluation of the first year of Pandora NO2 measurements over Beijing and application to satellite validation, Atmospheric Measurement Techniques Discussions, 2023, 1-32.
[https://doi.org/10.5194/amt-2023-177.inreview,2023.]
-
Ma, J.Z., Beirle, S., Jin, J.L., Shaiganfar, R., Yan, P., Wagner, T. (2013) Tropospheric NO2 vertical column densities over Beijing: results of the first three years of ground-based MAX-DOAS measurements (2008-2011) and satellite validation, Atmospheric Chemistry and Physics, 13, 1547-1567.
[https://doi.org/10.5194/acp-13-1547-2013]
- National Air Emission Inverntory and Research Center (NAIR) (2021) CAPSS. https://www.air.go.kr/capss/emission/sido.do?menuId=31
-
Newnham, D.A., Ballard, J. (1998) Visible absorption cross sections and integrated absorption intensities of molecular oxygen (O2 and O4), Journal of Geophysical Research: Atmospheres, 103(D22), 28801-28815.
[https://doi.org/10.1029/98JD02799]
-
Rothman, L.S., Gordon, I.E., Babikov, Y., Barbe, A., Benner, D.C., Bernath, P.F., Birk, M., Bizzocchi, L., Boudon, V., Brown, L.R., Campargue, A., Chance, K., Cohen, E.A., Coudert, L.H., Devi, V.M., Drouin, B.J., Fayt, A., Flaud, J.-M., Gamache, R.R., Harrison, J.J., Hartmann, J.-M., Hill, C., Hodges, J.T., Jacquemart, D., Jolly, A., Lamouroux, J., Le Roy, R.J., Li, G., Long, D.A., Lyulin, O.M., Mackie, C.J., Massie, S.T., Mikhailenko, S., Müller, H.S.P., Naumenko, O.V., Nikitin, A.V., Orphal, J., Perevalov, V., Perrin, A., Polovtseva, E.R., Richard, C., Smith, M.A.H., Starikova, E., Sung, K., Tashkun, S., Tennyson, J., Toon, G.C., Tyuterev, V.G., Wagner, G. (2013) The HITRAN2012 molecular spectroscopic database, Journal of Quantitative Spectroscopy and Radiative Transfer, 130, 4-50.
[https://doi.org/10.1016/j.jqsrt.2013.07.002]
-
Saiz-Lopez, A., Saunders, R.W., Joseph, D.M., Ashworth, S.H., Plane, J. (2004) Absolute absorption cross-section and photolysis rate of I2, Atmospheric Chemistry and Physics, 4(5), 1443-1450.
[https://doi.org/10.5194/acp-4-1443-2004]
-
Serdyuchenko, A., Gorshelev, V., Weber, M., Chehade, W., Burrows, J.P. (2013) High spectral resolution ozone absorption cross-sections - Part 2: Temperature dependence, Atmospheric Measurement Techniques Discussions, 6(4).
[https://doi.org/10.5194/amtd-6-6613-2013]
-
Shah, V., Jacob, D.J., Li, K., Silvern, R.F., Zhai, S., Liu, M., Lin, J., Zhang, Q. (2020) Effect of changing NOx lifetime on the seasonality and long-term trends of satellite-ob served tropospheric NO2 columns over China, Atmospheric Chemistry and Physics, 20(3), 1483-1495.
[https://doi.org/10.5194/acp-20-1483-2020]
-
Shaiganfar, R., Beirle, S., Sharma, M., Chauhan, A., Singh, R.P., Wagner, T. (2011) Estimation of NOx emissions from Delhi using Car MAX-DOAS observations and comparison with OMI satellite data, Atmospheric Chemistry and Physics, 11, 10871-10887.
[https://doi.org/10.5194/acp-11-10871-2011]
- Spietz, P. (2005) Absorption cross sections for iodine species of relevance to the photolysis of mixtures of I2 and O3 and for the atmosphere (Doctoral dissertation, Universität Bremen).
-
Thalman, R., Volkamer, R. (2013) Temperature dependent absorption cross-sections of O2-O2 collision pairs between 340 and 630 nm and at atmospherically relevant pressure, Physical Chemistry Chemical Physics, 15(37), 15371-15381.
[https://doi.org/10.1039/C3CP50968K]
-
Tzortziou, M., Herman, J.R., Cede, A., Abuhassan, N. (2012) High precision, absolute total column ozone measurements from the Pandora spectrometer system: Comparisons with data from a Brewer double monochromator and Aura OMI, Journal of Geophysical Research: Atmospheres, 117(D16).
[https://doi.org/10.1029/2012JD017814]
-
Vandaele, A.C., Hermans, C., Simon, P.C., Carleer, M., Colin, R., Fally, S., Merienne, M.-F., Jenouvrier, A., Coquart, B. (1998) Measurements of the NO2 absorption cross-section from 42 000 cm-1 to 10 000 cm-1 (238-1000 nm) at 220 K and 294 K, Journal of Quantitative Spectroscopy and Radiative Transfer, 59(3-5), 171-184.
[https://doi.org/10.1016/S0022-4073(97)00168-4]
-
Zhang, R., Tie, X., Bond, D.W. (2003) Impacts of anthropogenic and natural NOx sources over the U.S. on tropospheric chemistry, Proceedings of the National Academy of Sciences, 100(4), 1505-1509.
[https://doi.org/10.1073/pnas.252763799]
-
Zhang, X., Zhang, P., Zhang, Y., Li, X., Qiu, H. (2007) The trend, seasonal cycle, and sources of tropospheric NO2 over China during 1997-2006 based on satellite measurement, Science in China Series D: Earth Sciences, 50(12), 1877-1884.
[https://doi.org/10.1007/s11430-007-0141-6]
이기열 (한국외국어대학교 환경학과 석사과정) (lgy5848@hufs.ac.kr)
최용주 (한국외국어대학교 환경학과 조교수) (choingjoo@hufs.ac.kr)